FY2014 Annual Report
Developmental Neurobiology Unit
Associate Professor Ichiro Masai
Abstract
The vertebrate neural retina is derived from the ventral region of the forebrain. In this region, six classes of neurons differentiate and form the neural circuit underlying visual transduction. Thus, the retina provides an excellent model for studying cell differentiation and neural circuit formation in the vertebrate brain. Furthermore, more than one hundred hereditary retinal diseases causing photoreceptor degeneration have been identified in humans. Understanding the pathological processes of photoreceptor degeneration is an important issue from a medical perspective. We are currently investigating the mechanisms underlying retinal cell differentiation and photoreceptor degeneration, using zebrafish as an animal model. Regarding the project on retinal cell differentiation, in 2014, we showed that retinal neurogenesis is delayed in zebrafish stem-loop binding protein 1 (slbp1) mutant. Because SLBP1 regulates a replication-dependent synthesis and degradation of histone protein, this finding suggests that replication-coupled histone regulation is important for retinal neurogenesis. Furthermore, to examine cell-cycle regulation with high accuracy, we visualized cell-cycle phases using zebrafish fluorescent ubiquitination-based cell cycle indicator (Fucci). We applied this Fucci technique to zebrafish lens epithelium and revealed a spatial regulation of cell proliferation in zebrafish lens epithelium. Regarding the project on photoreceptor degeneration, in 2014, we examined zebrafish b-SNAP mutant showing photoreceptor degeneration. We showed that a BH3-only SNARE, BNip1, mediates photoreceptor apoptosis in absence of b-SNAP activity. We propose that BNip1 functions as an alarm that senses vesicular fusion defects and induces apoptosis.
1. Staff
- Dr. Yuko Nishiwaki, Group leader
- Dr. Toshiaki Mochizuki, Staff scientist
- Dr. Sachihiro Suzuki, Staff scientist (1 Sept 2014–)
- Dr. Maria Iribarne, Postdoctral scholar
- Mr. Yutaka Kojima, Technician
- Ms. Eri Oguri, Technician
- Mr. Masato Araragi, Technician
- Ms. Miyuki Suenaga, Technician
- Ms. Yayoi Tomoyose, Research Assistan
- Ms. Kazumi Toguchi, Research Assistant
- Ms. Moe Inafuku, Research Assistant
- Ms. Chitose Mizuta, Research Assistant
- Ms. Rui Inoue, Research Assistant (9 Mar 2015–)
- Ms Ayako Gima, Research Administrator/Secretary
2. Collaborations
- Theme: In vivo functional analysis of hypoxia response genes using the zebrafish retina
- Type of collaboration: Joint research agreement
- Researchers: Dr. Ichiro Masai (Developmental neurobiology unit, OIST), Dr. Masayuki Matsushita (Department of Medicine, Ryukyu University)
3. Activities and Findings
3.1 Mechanism regulating retinal neurogenesis in zebrafish
The vertebrate retina is initially specified in the anterior neural plate and evaginates from the ventral region of the forebrain as the optic cup. In this region, six major classes of retinal neurons and one class of glial cells differentiate to form the neural circuit that mediates phototransduction and visual processing. Thus, the retina provides an excellent model for studying the mechanisms underlying cell differentiation and neural circuit formation in the developing brain.
In the developing retina, retinal cells initially proliferate. At a particular developmental stage, retinal cells start to exit from cell cycle and differentiate into neurons, whose process is called neurogenesis. We have examined molecular mechanism that regulates retinal neurogenesis in zebrafish. Previously, we identified several signaling molecules, Hedgehog, Fgf, Wnt, Notch, Histone deacetylase 1, and a cell-polarity regulator MAGUK p55 superfamily number 5 (MPP5, zebrafish nagie oko), which cooperatively regulate the balance between proliferation and differentiation during retinal neurogenesis. However, molecular mechanisms that coordinate cell proliferation and neurogenesis are not fully understood.
We found that retinal neurogenesis is delayed in zebrafish stem-loop binding protein 1 (slbp1) mutant. SLBP1 binds to a stem-loop structure at the 3’-end of histone mRNAs, and regulates a replication-dependent synthesis and degradation of histone protein. We found that retinal cell proliferation becomes slow in the slbp1 mutant, resulting in cessation of retinal stem cell proliferation. Although retinal stem cells stop proliferation at 2 days post-fertilization (dpf), retinal progenitor cells in the central retina continue to proliferate and generate retinal neurons until 5 dpf (Fig. 1A). We found that this progenitor proliferation depends on Notch signaling, suggesting that Notch signaling maintains retinal progenitor proliferation when faced with reduced SLBP1 activity. Thus, SLBP1 cooperates with Notch singling to promote cell proliferation and subsequent neurogenesis in zebrafish retina (Fig. 1B). These data suggest that SLBP1 constitutes one of core components that regulate retinal neurogenesis.
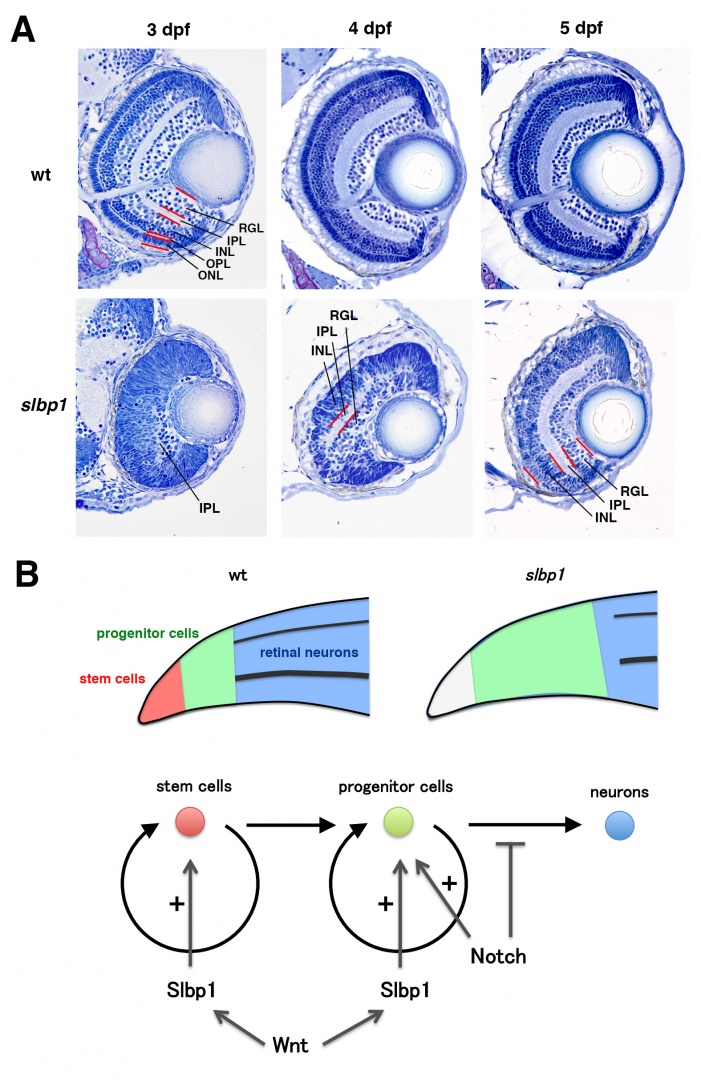
3.2 Visualization of cell-cycle phases with fluorescent proteins using Fucci
The first step of neurogenesis is the exit from the cell cycle. Previously we showed that the earliest neurogenic marker, ath5 (also designated as atoh7), is initiated in the G2 phase in retinal progenitor cells just prior to the final cell division, which generates two neuronal daughter cells. Thus, it is likely that the commitment to the retinal neuron is associated with cell-cycle progression. To accurately understand the relationship between cell proliferation and cell differentiation, we visualized cell-cycle phases differentially with fluorescent proteins, using the zebrafish Fucci (fluorescent ubiquitination-based cell cycle indicator) (Sugiyama et al., 2009, Proc Natl Acad Sci USA 106, 20812–20817). In the Fucci system, the cell-cycle dependent expression of two cell-cycle regulators, Geminin and Cdt1, are visualized with fluorescent proteins fused to their regulatory peptide sequences. We generated the zebrafish transgenic line carrying genes encoding mCherry-tagged Geminin and green fluorescent protein (GFP)-tagged Histone 2A. In this transgenic fish, we were able to clearly distinguish three different phases in the developing retina as well as the lens; the G1, S-G2, and M phases (Fig. 2A). Furthermore, we were able to observe chromosome condensation in the M phase (Fig. 2B) and measure the orientation of cell division from in vivo time-lapse imaging data.
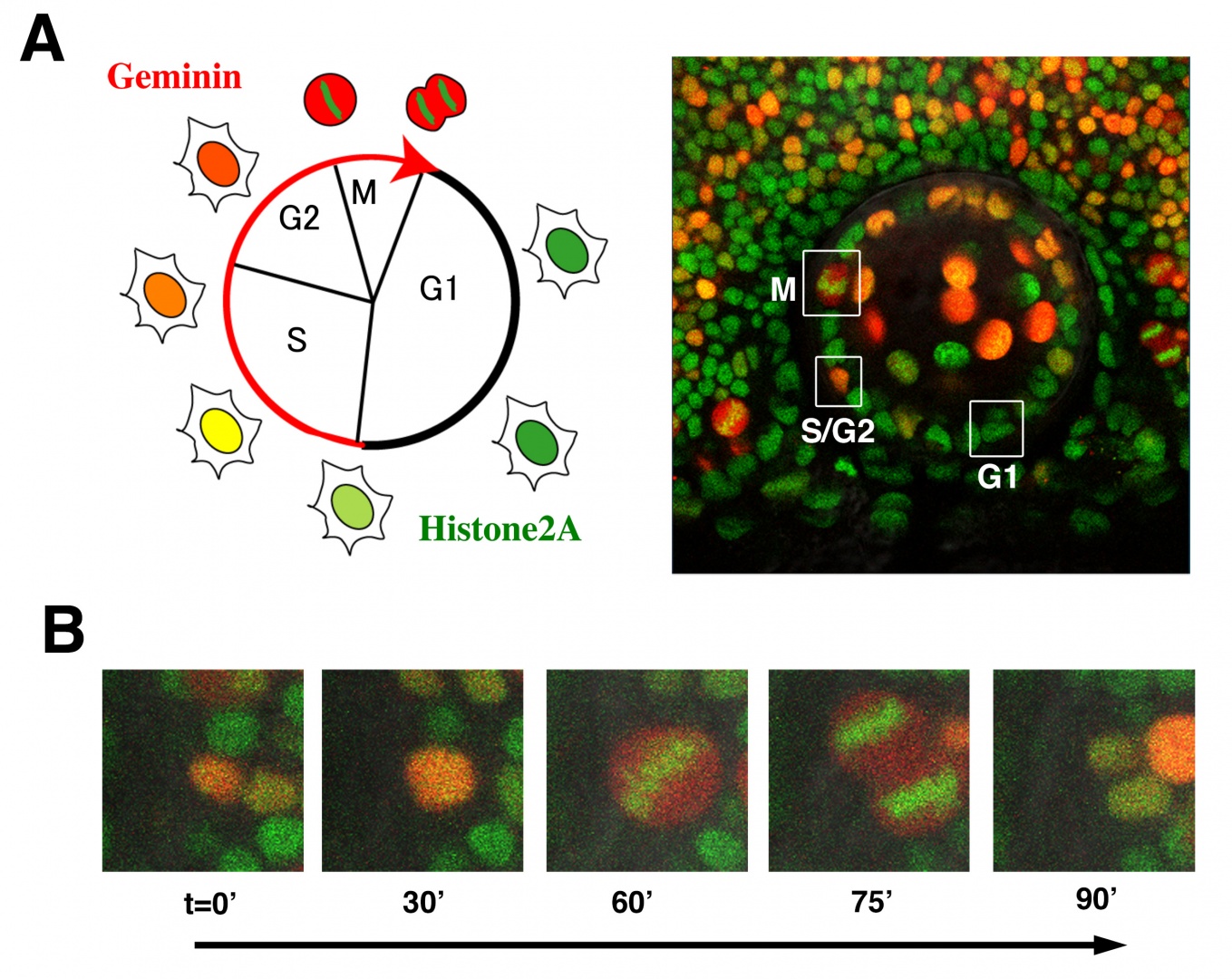
Using this transgenic fish, we observed the cell-cycle progression, cell division, and cell migration in the zebrafish lens epithelium using time-lapse techniques, because the lens epithelium is more easily accessed than the neural retina (Fig. 3A). We found that cell proliferation was highly active in a marginal zone just anterior to the equator in the lens epithelium. Furthermore, cell-division orientation was biased longitudinally in the anterior region of the lens epithelium, whereas it was circumferentially biased in the peripheral proliferating zone (Fig. 3B). These data suggest that cell proliferation and cell division are spatially patterned in the zebrafish lens epithelium (Fig. 3C). In many developing tissues, it has been reported that cells tend to divide along their long axis. This is referred to as “the Hertwig rule”. We found that the long axis was similarly biased longitudinally and circumferentially in the anterior and proliferating region in the zebrafish lens epithelium, respectively (Fig. 3D), suggesting that lens epithelial cells follow the Hertwig rule. These observations suggest that apical cell geometry correlates with cell division orientation in the lens epithelium.
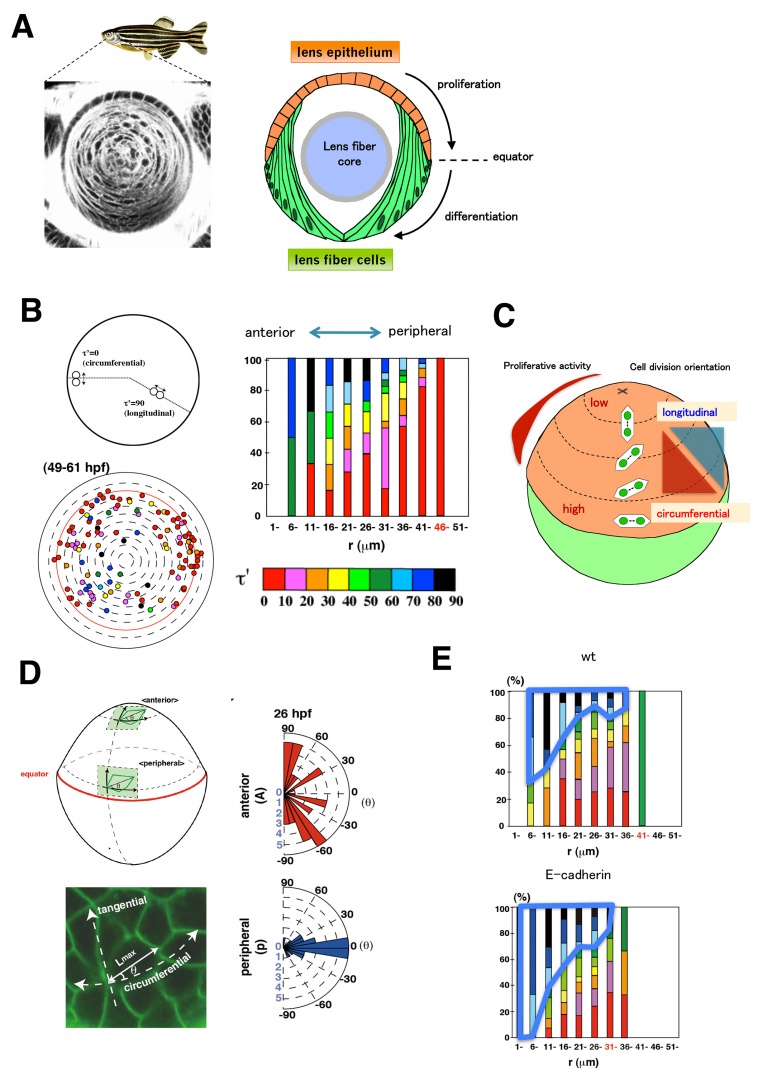
3.3 Mechanisms underlying photoreceptor degeneration
Photoreceptors are highly specialized neurons that efficiently detect light stimuli. To date, more than a hundred genes associated with inherited photoreceptor degeneration have been identified in humans (see the homepage of the Retinal Information Network at http://www.sph.uth.tmc.edu/Retnet/). However, the mechanisms underlying the pathological processes involved in photoreceptor degeneration remain to be elucidated. To determine the mechanisms underlying photoreceptor degeneration, we have screened zebrafish mutants with dysfunctional visual behavior by testing the optokinetic response. We identified several zebrafish mutants, in which photoreceptors differentiate but later degenerate. In 2010, we reported that one of these mutants carried a genetic mutation in cGMP-phosphodiesterase 6c (PDE6c), a mediator molecule of cone-specific phototransduction, suggesting a link between achromatopsia and progressive cone dystrophy. In 2012–2013, we examined another mutant, coa, whose photoreceptors rapidly undergo apoptosis before maturation. We found that the coa mutant gene encodes b-soluble N-ethylmaleimide-sensitive factor attachment protein (b-SNAP). b-SNAP regulates the fusion of transport vesicles to intracellular membrane organelles. These observations suggest that vesicular transport defects cause photoreceptor apoptosis in zebrafish.
Mitochondria-dependent apoptosis is promoted by pro-apoptotic Bcl2 family proteins, Bax, and inhibited by anti-apoptotic Bcl2 family proteins, Bcl2. BH3-only proteins promote apoptosis by modulating the balance between pro-apoptotic and anti-apoptotic Bcl2 proteins. We found that a BH3-only protein, BNip1, mediates the coa-mediated photoreceptor apoptosis. BNip1 is a component of the syntaxin 18 SNARE complex, and the syntaxin 18 SNARE complex regulates retrograde transport from the Golgi apparatus to the ER. b-SNAP promotes the recycle of vesicular fusion machinery, SNARE, by disassembling the cis-SNARE complex generated by vesicular fusion. Thus, it is likely that the syntaxin 18 cis-SNARE complex is accumulated in the coa mutant. We found that failed disassembly of the syntaxin 18 cis-SNARE complex activates the BNip1-dependent photoreceptor apoptosis in the coa mutant. These data suggest that the syntaxin 18 cis-SNARE complex monitors vesicular fusion competence and that BNip1 transforms vesicular fusion defects into apoptosis. From these data, we propose that BNip1 functions as an alarm that monitors abnormalities of vesicular fusion and transforms vesicular fusion defects into apoptosis in photoreceptors.
4. Publications
4.1 Journals
- Mochizuki, T., Suzuki, S., and Masai, I. Spatial pattern of cell geometry and cell-division orientation in zebrafish lens epithelium. Biol. Open 3, 982–994, doi: 10.1242/bio.20149563 (2014).
- Imai, F., Yoshizawa, A., Matsuzaki, A., Oguri, E., Araragi, M., Nishiwaki, Y. & Masai, I. Stem-loop binding protein is required for retinal cell proliferation, neurogenesis, and intraretinal axon pathfinding in zebrafish. Developmental biology, 391, 94-109, doi:10.1016/j.ydbio.2014.07.020 (2014).
- Mochizuki, T. & Masai, I. The lens equator: a platform for molecular machinery that regulates the switch from cell proliferation to differentiation in the vertebrate lens. Development, growth & differentiation 56, 387-401, doi:10.1111/dgd.12128 (2014).
4.2 Books and other one-time publications
Nothing to report
4.3 Oral and Poster Presentations
(Oral, International conference)
- Mochizuki, T., Luo, Y.-J., Suzuki, S., and Masai, I. . E-cadherin-dependent cell adhesion regulates cell migration pattern in the zebrafish lens epithelium, in 11th International Conference on Zebrafish Development and Genetics, Madison, USA (2014).
(Poster, international conferences)
- Nishiwaki, Y., Nakamura, S., Oguri, E., Araragi, M., Kojima, Y., and Masai, I. Molecular mechanism that converts vesicular fusion defects into apoptosis in photoreceptors, in 11th International Conference on Zebrafish Development and Genetics, Madison, USA (2014).
- Nishiwaki, Y., Nakamura, S., Oguri, E., Araragi, M., Kojima, Y. and Masai, I. Molecular Mechanism that converts vesicular fusion defects into apoptosis in photoreceptors, in Gordon research seminar – Visual System Development, Lucca, Italy (2014).
- Nishiwaki, Y., Nakamura, S., Oguri, E., Araragi, M., Kojima, Y. and Masai, I. Molecular mechanism that converts vesicular fusion defects into apoptosis in photoreceptors, in Gordon Research Conference –Visual System Development, Lucca, Italy (2014).
(Invited talks)
- Masai, I. Visualization of cell proliferation and migration in zebrafish lens epithelium, in Gordon Research Conference –Visual System Development, Lucca, Italy (2014).
(Oral, domestic conferences)
- Mochizuki, T., Luo, Y.-J., Suzuki, S., and Masai, I. E-cadherin-dependent cell adhesion regulates cell migration pattern in the zebrafish lens epithelium, in The 47th Annual Meeting of the Japanese Society of Developmental Biologists, Nagoya, Japan (2014).
- Nishiwaki, Y., Iribarne, M. and Masai, I. Genetic dissection of photoreceptor degeneration mechanism underlying zebrafish as a model for human hereditary retinal diseases, in The 20th Japanese Medaka and Zebrafish Meeting, Tokyo, Japan (2014).
- Suzuki, S. Planer patterning of cone photoreceptors in zebrafish retina, in The 7th Retina Research Meeting, Tokyo, Japan (2014).
(Poster, domestic conferences)
- Iribarne, M., Nishiwaki, Y. and Masai, I. Cone photoreceptor degeneration in zebrafish mutant gold rush, in The 47th Annual Meeting of the Japanese Society of Developmental Biologists, Nagoya, Japan (2014).
- Nishiwaki, Y., Oguri, E., Araragi, M., Nakamura, S., Yoshizawa, A., and Masai, I., Overexpression of ER-localized Bcl2 inhibits BNip1-dependent apoptosis, in The 37th Annual Meeting of the Molecular Biology Society of Japan, Yokohama, Japan (2014).
5. Intellectual Property Rights and Other Specific Achievements
Funding
KAKENHI (grants from the Ministry of Education, Science and Sport/JSPS)
Yuko Nishiwaki: Scientific research C (Kiban C) (2014–2016)
6. Meetings and Events
6.1 OIST course
- Title: Developmental Neurobiology Course 2014
- Co-organizer: Yoko Yazaki-Sugiyama (OIST), David L. van Vactor (Harvard Medical School, OIST), Gordon Arbuthnott (OIST)
- Dates: 29 June – 16 July 2014
- Place: OIST campus
- Speakers: David L. van Vactor (Harvard Medical School, OIST), Ichiro Masai (OIST), John Clarke (King’s College London), Tatushi Igaki (Kyoto University), Tako Hensch (Harvard University), Gordon Arbuthnott (OIST), Mineko Kengaku (Kyoto University), Anne Church Hart (Brown University), Keshav Dani (OST), Yoko Sugiyama (OIST), Yishi Jin (UC San Diego), Andrew Chisholm (UC San Diego), Ronald L. Davis (Scripps Research Institute Florida), Gina Turrigiano (Brandeis University), Sacha Nelson (Brandeis University), Masashi Yanagisawa (University of Tsukuba), Yoshihiro Yoshihara (RIKEN BSI), Atsushi Miyawaki (RIKEN BSI), Aravinthan Samuel (Harvard University), Hiroshi Kohsaka (University of Tokyo), Hiroshi Kawasaki (Kanazawa University), Thomas A Reh (University of Washington Seattle), Yasunori Hayashi (RIKEN BSI), Lee Rubin (Harvard University)
6.2 OIST mini-symposium
- Title: Microglia: key to understand neural development and pathlogy”
- Co-organizer: Sumiko Watanabe (University of Tokyo), Akira Murakami (Juntendo University Graduate School of Medicine)
- Dates: 27 February – 1 March 2015
- Place: OIST campus
- Speakers: Ichiro Masai (OIST), Sumiko Watanabe (University of Tokyo), Francesca Peri (EMBL, Heidelberg, Germany), Kazuhide Inoue (Kyusyu University), Akira Murakami (Juntendo University Graduate School of Medicine), Wen-biao Gan (New York University School of Medicine), Michal Schwartz (Weizmann Institute), Dongsheng Cai (Albert Einstein College of Medicine), Jiulin Du (CAS Center for Excellence in Brain Science), Hideo Kohno (Jikei University School of Medicine), Wai T. Wong (National Eye Institute, NIH), Takayuki Harada (Tokyo Metropolitan Institute of Medical Science),
6.3 OIST seminar
(1) Speaker: Dr. Yumiko Yamazaki-Kato
Affiliation: Graduate school of science, Hokkaido University
Title: “Analysis of the function of miR-124 during medaka neural development”
Date: May 8, 2014
(2) Speaker: Dr. Sachihiro Suzuki
Affiliation: Department of Biological Structure, University of Washington
Title: “Generation of functionally distinct cone photoreceptor types in zebrafish”
Date: May 15, 2014
(3) Speaker: Dr. Noriko Yutsudo
Affiliation: Kobe Pharmaceutical University
Title: “fosB-null mice display impaired adult hippocampal neurogenesis and spontaneous epilepsy with depressive behavior”
Date: May 19, 2014
(4) Speaker: Dr. Hongping Wei
Affiliation: Institute of Neuroscience, Shanghai Institute for Biological Science, Chinese Academy of Science
Title: “Long-term synaptic plasticity in zebrafish retina”
Date: Sept 17, 2014
(5) Speaker: Dr. Takeshi Iwata
Affiliation: Division of Molecular and Cellular Biology, National Institute of Sensory Organs Tokyo Medical Center, National Hospital Organization
Title: “Genes and Molecular Mechanism of Retinal Diseases”
Date: Jan 13, 2015
(6) Speaker: Dr. John McAvoy
Affiliation: Save Sight Institute, Sydney Hospital and Eye Hospital, University of Sydney
Title: “A network of growth factor signaling determines lens polarity and its precise three-demensional cellular architecture”
Date: Feb 20, 2015
(7) Speaker: Dr. Frank J. Lovicu
Affiliation: School of Medicine, Save Sight Institute and Bosh Institute, University of Sydney
Title: “Negative regulations of growth factor signaling in the lens”
Date: Feb 20, 2015
7. Other
Nothing to report.