FY2021 Annual Report
Marine Climate Change Unit
Professor Timothy Ravasi
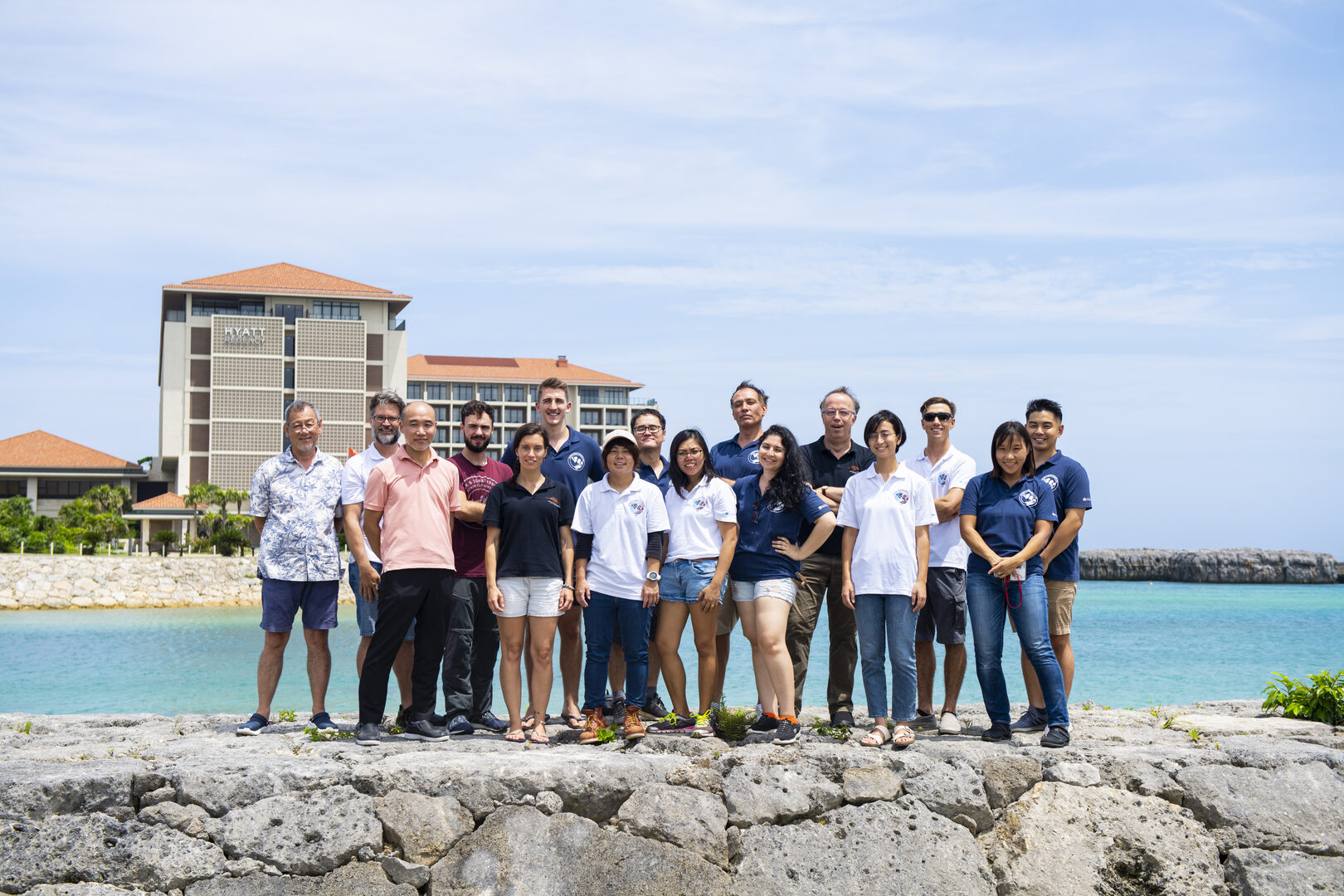
Abstract
Our unit aims to understand how coral reef fish adapt to environmental changes such as climate change, heatwaves, overfishing and urbanization. Earth's oceans are warming and acidifying due to increasing anthropogenic CO2 production, and extreme events such as marine heatwaves are increasing in frequency, duration, and magnitude. Coral reef fish are especially vulnerable to spikes in temperature because they are already adapted to living close to their thermal limits. Determining which species can adapt to rapid environmental change, and how they will do so, is critical for predicting the ecological effects of climate change and their impact on the world economy.
In our laboratory research, we investigate how short-term as well as heritable changes in gene expression in multiple fish species are affected by increases in sea level temperature and acidity. We study animals that are descended from wild breeding pairs and have been reared in our aquariums over several generations. Using a unique device called the Heatwaves Simulator aquarium system based at OIST’s Marine Science Station, we expose fish to specific conditions, including the temperature and acidity level predicted to occur in seawater by the end of the century. We combine these manipulations with a range of advanced genomic technologies to identify the cellular mechanisms that allow coral reef fish communities in Okinawa and around the world to acclimate and adapt to changing conditions.
1. Staff
- Tae Woo Ryu, Research Unit Group Leader
- Roger Huerlimann, Postdoctoral Scholar
- Shannon McMahon, Postdoctoral Scholar
- Erina Kawai, Research Unit Scientific Diver
- Jeffrey Jolly, Research Unit Technician
- Chengze Li, Research Unit Technician
- Radmila Neiman, Fish Husbandry Technician
- Gelyn Bourguignon, Fish Husbandry Technician
- Michael Izumiyama, Graduate Student
- Billy Moore, Graduate Student
- Ayşe Haruka Oshima Açıkbaş, Graduate Student
- Kaisar Dauyey, Special Research Student
- Jodi Thomas, Visiting Research Student
- Naomi Asato, Research Intern
- Johanna Linnea Johansson, Research Intern
- Yoko Shintani, Research Unit Administrator
- Jann Zwahlen, Rotation Student
- Sourjya Baibhabee Nath, Rotation Student
2. Collaborations
2.1 Anemonefish development and genomics
- Type of collaboration: Scientific Collaboration
- Researcher:
- Professor Vincent Laudet, OIST, Japan
2.2 Coral Reef fish adaptation to marine heatwaves
- Type of collaboration: Scientific Collaboration
- Researchers:
- Professor Philip Munday, James Cook University, Australia
- Professor Moisés A. Bernal, Auburn University, USA
2.3 Coral Reef fish adaptation to Ocean Acidification
- Type of collaboration: Scientific Collaboration
- Researcher:
- Professor Celia Schunter, The University of Hong Kong, HongKong
2.4 Coral Reef fish genomics
- Type of collaboration: Scientific Collaboration
- Researchers:
- Deputy Director Piero Carninci, RIKEN Center for Integrative Medical Sciences, Japan
- Professor Jesper Tegnér, KAUST, Saudi Arabia
- Professor Valerio Orlando, KAUST, Saudi Arabia
2.5 Coral Reef fish Physiology
- Type of collaboration: Scientific Collaboration
- Researcher:
- Professor Martin Grossel, University of Miami, USA
2.6 Impact of coastal development on coral reef ecosystems
- Type of collaboration: Scientific Collaboration
- Researchers:
- Professor Jose M. Eirin-Lopez, Florida International University, USA
- Professor James Davis Reimer, University of Ryukyus, Japan
- Professor Noriyuki Satoh, OIST, Japan
2.7 Natural analogues of climate stressors
- Type of collaboration: Scientific Collaboration
- Researcher:
- Dr. Riccardo Rodolfo-Metalpha, UMR ENTROPIE, Nouvelle Calédonie
2.8 Natural Volcanic CO2 Analogues
- Type of collaboration: Scientific Collaboration
- Researchers:
- Professor Ivan Nagelkerken, The University of Adelaide, Australia
- Professor Sylvain Agostini, University of Tsukuba, Japan
2.9 Okinawa Coral spawning and development
- Type of collaboration: Scientific Collaboration
- Researcher:
- Professor David J. Miller, James Cook University, Australia
2.10 Sharks physiology under climate stressors
- Type of collaboration: Scientific Collaboration
- Researchers:
- Professor Jodie L. Rummer, James Cook University, Australia
- Professor Rui Rosa, Faculdade de Ciências da Universidade de Lisboa, Portugal
2.11 Transgenerational acclimation
- Type of collaboration: Scientific Collaboration
- Researcher:
- Dr. Jennifer Donelson, James Cook University, Australia
3. Activities and Findings
3.1 Design and Construction of the OIST Heatwaves Simulator aquariums system.
This year our unit in collaboration with Luxaqua, we designed and constructed the OIST Heatwaves Simulator, a unique aquariums system that will allows us and our collaborators to recreate a future environment including the simulation of marine heatwaves at the OIST Marine Science Station.
The OIST Heatwaves Simulator consist of three separate tank systems (Figure 1); 1) 10, 100L outdoor breeding pair tanks that will be used to house brood stock breeding pairs, 2) 10, 75L larval rearing tanks, that from 2022 onwards (F2, F3) will be used in the larval rearing stage of this multi-generational experiment, 3) 144, 55L tanks that will house experimental juvenile fish in different treatments. Temperature within these experimental tanks is controlled by four inline heaters, that allow five different temperature treatments to be specified in each individual tank. Temperature is controlled and monitored by automated software and associated temperature probes. Software also has remote monitoring and control capabilities.
This system is unique in the world and it will help to incentive collaborators from Japan and overseas to come to OIST and work with us at the marine station.
Figure 1. Schematic (A) and 3D floor plan (B) of the aquaria system being installed at OIST marine science station in June/July 2021. Aquaria will consist of 144, 55L tanks (B; blue box), 10, 75L larval rearing tanks (B; red box) and 10, 100L outdoor breeding pair tanks (B; orange box).
3.2 Coral Reef fish Physiology and Metabolic Performance
To assess the effects of the different heatwave treatments on coral reef fish’s metabolic rates, in collaboration with Loligo Systems, we set up at the OIST Marine Station a 170ml mini-swim tunnel (Figure 1). Water flow within the swim tunnel is generated by a propeller, and laminar flow is created by a series of flow straightening plastic honeycomb meshes. The velocity of water within the tunnel is controlled by Loligo Systems AutoResp software and is calibrated using Loligo Systems fluorescent PE microspheres and the associated Digital Particle Tracking Velocity software. An oxygen dipping probe is inserted into the swim tunnel, allowing for continuous measurements of oxygen concentration within the tunnel. A pump can be used to flush the swim tunnel with fresh seawater from the waterbath if required. The swim tunnel is submerged within a temperature controlled waterbath allowing the temperature within the swim tunnel to be manipulated. Flushing of the swim-tunnel and resting chambers, monitoring of oxygen concentrations and temperature, and calculations of oxygen consumption rates are all completed automatically by Loligo Systems AutoResp software.
Figure 2. (A) Schematic of one Loligo Systems resting chamber set-up used to quantify RMR. A pump (A1) is used to flush the chamber with fresh seawater from the waterbath. A pump (A2) is used to recirculate water through a closed loop that passes through a flow through oxygen cell, within which oxygen concentration is measured by a fibre optic oxygen sensor (A3). (B) Schematic of a Loligo Systems 170ml mini-swim tunnel used to quantify MMR. A pump (B1) is used to flush the swim tunnel with fresh seawater from the waterbath. Water flow is generated within the swim tunnel by a propeller (B2) and oxygen concentration is measured by a 200mm oxygen dipping probe inserted into the opposite end of the tunnel (B3).
3.3 Amphiprion ocellaris Spawning and Larval Rearing
In order to conduct the planned long-term multi-generational experiment detailed above, we first needed to ensure that wild-caught A.ocellaris from Okinawan coral reef systems would spawn within aquaria. To test this, one breeding pair of A.ocellaris was acquired from local fishermen in September 2019. This pair was placed within a 200L aquaria at OIST Marine Science Station with a sea anemone (Heteractis magnifica) and multiple rocks.
Figure 3. Images displaying (a) fertilized eggs of A.ocellaris (b) larval rearing tank and (c) juvenile A.ocellaris 1 month after hatching within aquaria at OIST marine science station.
On the 31st of May 2020 eggs were observed within the breeding pair tank (Figure 1). The development of eggs was tracked using visual observation and comparisons to know stages of embryonic development. On the 7th of June, eggs were transferred to a larval rearing tank for hatching (Figure 3) and larval A.ocellaris were present in the tank on the 8th of June.
The successful spawning and larval rearing program of 2020 allowed us to develop for the first time at OIST Marine Science Station a larval rearing protocol.
3.4 The Sequencing of Amphiprion ocellaris, Amphiprion clarkii and Epinephelus malabaricus genomes.
Our unit sequenced the genomes of three coral reef fish from the water of Okinawa island, the Amphiprion ocellaris, Amphiprion clarkii and Epinephelus malabaricus (Table 1). All these genomes have been sequenced using PacBio long-reads sequencing combined with Illumina gDNA and transcriptome sequencing and binned into chromosomes using Hi-Seq contact map technology.
These genomes are among the most completed fish genomes ever sequenced and are available free for the entire community.
Table 1. Final assembly statistics for chromosome scale A.ocellaris and A. Clarkii genomes.
#Chronosomes | Total BP | # of Bases | GC Content | Coverage | Genes |
24 | 856,535,073 |
N: 134,893 A: 258,699,708 C: 169,470,711 G: 169,494,040 T: 258,735,721 |
39.58% | 103 X | 26,112 |
Assembly |
Number of Contigs |
Total Length | N50 | Largest Contig | Metazoa BUSCO | Eukaryote BUSCO | Actinopterygii BUSCO |
FALCON UNZIP | 1283 | 848,397,549 | 932,534 | 8,021,000 |
C: 98.5% S: 96.3% D: 2.2% |
C: 98.1% S: 95.7% D: 2.4% |
C: 96.7% S: 95.2% D: 1.5% |
Figure 4. Synteny map between A.ocellaris and the clownfish Amphiprion percula. Generated using orthologous genes from the A.ocellaris genome sequenced here, and the A.percula genome sequenced by Lehmann et al. (2019). Figure generated by Marcela Herrera Sarrias.
3.5 Natural Analogues of Future Climate
Unique sites which are natural analogues of future ocean have a community that already exists under this naturally occurring extreme environment and can be utilized as a natural laboratory. These sites are subjected to daily changes in ocean chemistry, have dynamic interactions between habitat, predator, and prey, and have existed in long timescales. Inhabitants may have also adapted to these extreme conditions through generations. Although conditions at each extreme site vary and are not perfect simulations of future oceans, they can provide a window into how species may respond to climate change.
Our unit successful performed field expeditions at the following natural analogues.
Bourake, New Caledonia
In the spring of 2020, with the collaboration of the IRD and several other universities, we conducted a two-week expedition to Bourake, New Caledonia, where we collected four species of fishes (Dascyllus aruanus, Zoramia leptacantha, Chaetodon lunulatus, Pomacentrus molluscensis) from future and control sites.
Figure 5. Map of Bourake, New Caledonia. A. The red dots represent the lagoon (future condition) where hot deoxygenated water flows during the outgoing tide. Blue dots represent control reefs (current condition) B. Photos of the lagoon.
Ioutorijima, Japan
In the fall of 2020, our team and collaborators from Tokyo University and the University of the Ryukyus conducted a three-day trip to the uninhabited island Ioutorijima. We collected eDNA samples and placed baited remote underwater cameras (BRUV) at the CO2 seep. We collected eDNA samples from the seep (future) and a nearby reef (control). We placed BRUVs with krill to determine the species that were present at the seep. Additionally, this trip produced a publication led by Reimer et al. (2021), which discovered and described a rare occurrence of aragonite-forming Nanipora at the CO2 seep site.
Figure 6. Map of Ioutorijima, Okinawa. The red circle indicated the CO2 Seep site, and the blue circle indicates the control reef. Water samples were collected at the yellow marker at the control site and the star located at the seep site.
Figure 7. Underwater photos of the habitat at CO2 Seep site (left) and Control reef (right). The bubbles are CO2 seeping from the ground.
Shikine island, Japan
In December 2020, our team conducted preliminary work for our project at Shikine island, Japan. Water samples for eDNA analysis were collected from the seep and control site. We also conducted a visual survey of the seep and control site to determine the species we will collect for our future study. The seep site was dominated by turf algae and had many herbivores similar to Ioutorijima.
The third image, Figure 3, is a single image with the caption below it.
Figure 8. Map of Shikine island and an expanded view of both control and seep site. The seep site is located at the top, and the control reef is at the bottom of the map. The blue dots represent where we collected water samples for eDNA analysis.
Figure 9. Underwater image of CO2 seep (left) control reef (right) at Shikine island. The CO2 seep site was covered in turf algae compared to the control reef, dominated by coral.
3.6 Tropicalizing Range-Shifts in the Japanese Coastlines via Environmental DNA Metabarcoding (eDNA).
Since the industrial age, anthropogenic activities have resulted in a consistent increase of sea surface temperature and ocean acidification, causing a world-wide shuffling of species, as part of an overall poleward range shift in both terrestrial and aquatic ecosystems. Specifically for coral reef recruitment, a tropical decrease and subtropical increase at the 20° latitude have been recorded with shifts to the more temperate areas of Southern Japan. This “tropicalization” raises the questions whether the Ryukyu arc islands could be experiencing this poleward shift along its axis (taking into account the effect of the Kuroshio current) and if it is also acting as a refugia for the migrating coral reef communities from near the equator.
Are the high latitude coral communities in Okinawa and Southern Japan the future areas of refuge for the range-shifting reef organisms, with the help of Kuroshio? Even if so, how would all the coastal development taking place in Okinawa affect this in the short-term and how immediately disruptive it could be? Could these migrating organisms be fitter compared to the original residents and what implications does this have for the future biodiversity of Okinawa? And how could the climate change be affecting the speciation process in the long-term, when compared to the past drastic changing phases in the climate?
The current climate-driven range shifts and therefore the opening-up of novel niches could serve as a window to see into and characterize the process of speciation with hybridization and adaptive radiation. Searching for and studying these newly created potential hybrid zones, we could gain a better understanding of speciation mechanisms in a changing and connected ecosystem. This hybrid swarm theory of adaptive radiation could be tested using population genetics and transcriptomics, in the nature and in the laboratory using the clownfish model organism.
In an increasingly warm world, it is crucial to understand how the changing conditions are affecting the species for more effective mitigation and conservation efforts. Effective design and management of MPAs can be achieved using the information gained from population connectivity and genomics studies, which can point to important sites of refuge/recruitment/nurseries. Temporal and spatial sampling for and studying eDNA across Okinawa and Japan can characterize the effects of the immediately disruptive anthropogenic activities such as coastal development and reconfiguration on biodiversity.
Figure 10. Marine Climate Change Unit members collecting and filtering seawater for environmental DNA (eDNA) studies in several location across Japan.
4. Publications
4.1 Journals
- Rapid evolution fuels transcriptional plasticity to ocean acidification. J Kang, I Nagelkerke, JL Rummer, R Rodolfo-Metalpa, PL Munday, C Schunter, T Ravasi. Global Change Biology. 2022, in press (DOI: 10.1111/gcb.16119).
- Molecular responses of brains to cross-generational warming in a coral reef fish. MA Bernal, E Schmidt, J Donelson, P Munday, T Ravasi. Frontiers of Marine Science 9:784418.
- A chromosome-scale genome assembly of the false clownfish, Amphiprion ocellaris. T Ryu, M Herrera, B Moore, M Izumiyama, E Kawai, V Laudet, T Ravasi. bioRxiv. 2022.
- Plasticity to ocean warming is influenced by transgenerational, reproductive, and developmental exposure in a coral reef fish. MA Bernal, T Ravasi, GG Rodgers, PL Munday, JM Donelson. Evolutionary Applications, 2021, https://doi.org/10.1111/eva.13337.
- Molecular basis of parental contributions to the behavioural tolerance of elevated pCO2 in a coral reef fish. AA Monroe, C Schunter, MJ Welch, PL Munday, T Ravasi. Proceedings of the Royal Society B 288 (1964), 202119312021, 2021
- Diel pCO2 fluctuations alter the molecular response of coral reef fishes to ocean acidification conditions. C Schunter, MD Jarrold, PL Munday, T Ravasi. Molecular Ecology 30 (20), 5105-5118, 2021.
- The time course of molecular acclimation to seawater in a euryhaline fish. LC Bonzi, AA Monroe, R Lehmann, ML Berumen, T Ravasi, C Schunter. Scientific reports 11 (1), 1-16, 2021.
- Sex‐and time‐specific parental effects of warming on reproduction and offspring quality in a coral reef fish. RK Spinks, LC Bonzi, T Ravasi, PL Munday, JM Donelson. Evolutionary Applications 14 (4), 1145-1158, 2021.
- Unexpected high abundance of aragonite-forming Nanipora (Octocorallia: Helioporacea) at an acidified volcanic reef in southern Japan. JD Reimer, H Kurihara, T Ravasi, Y Ide, M Izumiyama, H Kayanne. Marine Biodiversity 51 (1), 1-5, 2021.
- Neuro-molecular characterization of fish cleaning interactions. S Ramírez-Calero, JR Paula, E Otjacques, R Rosa, T Ravasi, C Schunter. bioRxiv, 2021.
- Desert fish populations tolerate extreme salinity change to overcome hydrological constraints. C Schunter, LC Bonzi, J Norstog, J Sourisse, ML Berumen, Y Angel, Parkes SD, McCabe MF, Ravasi T. bioRxiv, 2021.
- The role of ligand-gated chloride channels in behavioural alterations at elevated CO2 in a cephalopod. Jodi T. Thomas, Blake L. Spady, Philip L. Munday, Sue-Ann Watson, Journal of Experimental Biology 224 (13): jeb242335. doi.org/10.1242/jeb.242335
- Phylogenomics illuminates the evolution of bobtail and bottletail squid (order Sepiolida), Gustavo Sanchez, Fernando Á. Fernández-Álvarez, Morag Taite, Chikatoshi Sugimoto, Jeffrey Jolly, Oleg Simakov, Ferdinand Marlétaz, Louise Allcock & Daniel S. Rokhsar, Communications Biology 4: 819 (2021) doi.org/10.1038/s42003-021-02348-y
- Rapid multi-generational acclimation of coralline algal reproductive structures to ocean acidification. Moore, B., Comeau, S., Bekaert, M., Cossais, A., Purdy, A., Larcombe, E., Puerzer, F., McCulloch, M.T. and Cornwall, C.E. Proceedings of the Royal Society B, 288:20210130.
- Two temperate corals are tolerant to low pH regardless of previous exposure to natural CO2 vents. Carbonne, C., Teixidó, N., Moore, B., Mirasole, A., Guttierez, T., Gattuso, J.P. and Comeau, S. Limnology and Oceanography, 66(11), pp.4046-4061
- Improved detection sensitivity using an optimal eDNA preservation and extraction workflow and its application to threatened sawfishes. Cooper MK., Huerlimann, R., Edmunds, R. C., Budd, A. M., Le Port, A., Kyne, P. M., Jerry, D. R.,Simpfendorfer, C.A. Aquatic Conservation 31(8) pp.2131-2148
- First detection of critically endangered scalloped hammerhead sharks (Sphyrna lewini) in Guam, Micronesia, in five decades using environmental DNA. Budd, A. M., Cooper, M. K., Le Port, A. Schils, T., Mills, M. S., Deinhart, M. E., Huerlimann, R., Strugnell, J. M. Ecological Indicators 127: 107649.
- Using bacterial whole genome sequencing to identify toxin genes associated with disease outbreaks in black tiger shrimp (Penaeus monodon) aquaculture production. Condon, K., Huerlimann, R., Charles, T., Lowrey, R., Arbon, P., Jerry, D. R. Aquaculture 546: 737255.
- Digital Droplet PCR-Based Environmental DNA Tool for Monitoring Cryptocaryon irritans in a Marine Fish Farm from Hong Kong. Hin Hung Tsang,Jose A. Domingos, Jacob A. F. Westaway, Maximilian H. Y. Kam, Roger Huerlimann and Giana Bastos Gomes Diversity 2021, 13(8), 350.
4.2 Books and other one-time publications
Nothing to report
4.3 Oral and Poster Presentations
- Timothy Ravasi, “The Coral Reefs of Okinawa”. Climate Change and Blue Economy Seminar. The Japan Society, New York City, USA. February 2, 2022.
- Timothy Ravasi, Discussion Leader at the OIST-Tohoku University Joint Workshop on Marine Sciences – Climate Change. Okinawa, January 22, 2022.
- Timothy Ravasi,“Adaptation and Acclimation of Coral Reef Fish as a Response to Climate Change “. Universidad de Santiago de Compostela, Spain, January 14, 2022.
- Timothy Ravasi, “The OIST Marine Science Station”. World Congress of Marine Stations. University of Edinburgh, UK Polar Network (UKPN), November 17, 2021.
- Timothy Ravasi, “Evidence of local adaptation to acidifying oceans”. ICONA-JSPS Kick-off Symposium: International CO₂ & Natural Analogues Network. Shimoda Marine Science Center, University of Tsukuba, September 7th, 2021.
- Jodi Thomas, Roger Huerlimann, Timothy Ravasi, et al. "Transcriptomic and behavioural responses of a cephalopod to elevated CO2". Physiomar 2021 Online Conference, September 7, 2021.
- Jodi Thomas, "Rising CO2 levels affect the ‘inkredible’ squid brain", Three Minute Thesis Virtual Asia-Pacific Semi-Finals 2021, September 16, 2021.
- Billy Moore, "Rapid multi-generational acclimation of coralline algal reproductive structures under ocean acidification", 14th ICRS 2021, September 19, 2021
- Billy Moore, "Impacts of ocean warming on clownfish larvae", Okinawa Marine Science Workshop 2021, November 22, 2021
5. Intellectual Property Rights and Other Specific Achievements
Nothing to report
6. Meetings and Events
- [Seminar] Rise of the turfs: the simplification of marine ecosystems under ocean acidification by Dr. Ben Harvey, Tsukuba University
7. Media outreach
- Featured in Science Portal, science news media run by JST: 「海洋熱波で熱帯魚に生理学的影響 沖縄科学技術大学院大学などが解明」https://scienceportal.jst.go.jp/newsflash/20200327_02/
- Featured in web media OKINAWA 41 run by cabinet office: 「美しいサンゴや色とりどりの熱帯魚が壊滅的な被害!?その原因を紐解く」https://www.okinawa41.go.jp/reports/37253/
- Featured in Asahi Shimbun Globe+: 「海の酸性化が進むとどうなるか? 沖縄の無人島近くの海底で見つけたヒント」https://globe.asahi.com/article/13881750
- Hyatt Hotels News: OIST の海洋気候変動ユニットとはhttps://hyattregencyseragaki.jp/activity/kumanomi_news/001
- Research into squid no game for student, Townsville bulletin, November 24, page 2
7. Educational outreach
- Lecture by Erina Kawai, "Life of field work technician in Okinawa", at online event Lecture of vocation, OIST and Okinawa at Katsushika sogo high school
- Presentation by Radmila Neiman, "introducing marine life" to OIST Child Development Center: Kaffu, Miyarabi, Wakatida Classes, March 2022
- Presentation by Radmila Neiman, OIST fondation tour, March 11, 2022