FY2016 Annual Report
Micro/Bio/Nanofluidics Unit
Professor Amy Shen
Abstract
In FY2016, Micro/Bio/Nanofluidics Unit (MBNU) continued the 2 key research themes: one is related to the fundamental aspects of hydrodynamics, fluid manipulations, and flow instabilities in various microfluidic platforms (i.e., cross-slot, T-junction, flow focusing), with both Newtonian and non-Newtonian fluids. Another thrust is to use micro- and nanofluidics and nanofabrications for biotechnology applications, with emphasis on immunoassays and LSPR biosensing. For example, micro and nano-contact printing has been integrated in microfluidic devices for multiplexed immunoassay reactions. We also initiated new research directions in LSPR based biosensing, taking advantage of novel nanomaterials and new sensing techniques. Members of the MBNU unit published 14 peer-reviewed papers in FY 2016, with our research highlighted in R&D Magazine, Science Daily and such.
The unit members have also actively disseminated our research results to the general public and scientific communities. We have given a total of 20 presentations and seminars in FY 2016, while participating core professional society meetings such as the Society of Rheology Annual Meeting, micro-TAS, ACS Colloids and Surface Science Meeting, APS-DFD Annual meeting, and AICHE Annual meeting. Our unit sponsored activity "WALKING ON WATER" was again a huge success at the Annual Open Campus event at OIST.
Our unit organized a successful OIST mini-symposium titled "Science and Technology at the Interface of Bio-Nano-systems: Challenges and Opportunities” in April 2016. In addition, our unit organized a successful OIST workshop in August 2016, titled "New Aspects of Micro- and Macro-scopic Flows in Soft Matters". We also hosted 19 internationally renowned scientists visiting OIST and giving research seminars in FY 2016, with topics ranging from microfluidics, interfacial science, and novel materials.
Personnel wise, we have been actively recruiting new members. Besides the 3 Ph.D students, Mr. Hsieh-Fu (Paul) Tsai, Ms. Shivani Sathish, and Ms. Noa Burshtein recruited in FY 2015, the unit also recruited 1 new technician, Mr. Kei Funakoshi. Both Noa and Paul received JSPS DC1 fellowship in 2016. Our unit also welcomed a JSPS Postdoc Fellow, Dr. Mandy Leung from the University of Adelaide, Australia. Former postdoc, Dr. Doojin Lee, took a great position as a Senior Researcher at Korea Institute of Ceramic Engineering and Technology, Korea. Our unit also hosted 3 rotation students and 4 research interns from University of Leeds, McGill University, University of Hawai'i at Manoa and Stanford University.
1. Group Members
As of March 31, 2017
- Prof. Amy Shen, Professor
- Dr. Simon Haward, Group Leader
- Dr. Casey James Galvin, Postdoctoral Scholar
- Dr. Francesco Del Giudice, Postdoctoral Scholar
- Dr. Nikhil Bhalla, Postdoctoral Scholar
- Dr. Mandy Leung, JSPS Postdoctoral Scholar
- Mr. Kazumi Toda-Peters, Technician
- Mr. Kei Funakoshi, Technician
- Mr. Hsieh-Fu Tsai, Graduate Student
- Ms. Shivani Sathish, Graduate Student
- Ms. Noa Burshtein, Graduate Student
- Ms. Ainash Garifullina, Rotation Student
- Ms. Afshan Jamshaid, Rotation Student
- Mr. Agneesh Barua, Rotation Student
- Ms. Yuno Kaneshi, Research Administrator
Alumni
- Dr. Doojin Lee, Postdoctoral Scholar
- Mr. Alexander Unterkreuter, Technician
- Mr. Tsung-Han Hsieh, Graduate Student (Rotation)
- Mr. Sergey Zobnin, Graduate Student (Rotation)
- Mr. Rintaro Hayashi, Research Intern (Back to the University of Hawai'i at Manoa)
- Mr. Abhishek Sinha, Research Intern (Back to McGill University, Canada)
- Mr. Mohmed Ashra Mulla, Research Intern (Back to the University of Leeds)
- Ms. Mariana Hakim, Reserach Intern (Back to Stanford University, USA)
2. Collaborations
2.1 Flow instabilities in microfluidics
- Type of collaboration: Joint research
- Researchers:
- Professor Gerry Fuller, Stanford University, USA
- Professor Gareth McKinley, MIT, USA
- Professor Tamer Zaki, Johns Hopkins University, USA
- Professor Amy Shen, OIST
- Dr. Simon Haward, OIST
- Dr. Doojin Lee, OIST
2.2 Micro- and bulk rheology measurements of complex fluids
- Type of collaboration: Joint research
- Researchers:
- Professor Manlio Tassieri, University of Glasgow, UK
- Dr. Claude Oelschlae, KIT, Germany
- Professor Amy Shen, OIST
- Dr. Francesco Del Giudice, OIST
- Dr. Simon Haward, OIST
2.3 Micro and Nanofluidics for Biotechnology applications
- Type of collaboration: Joint research
- Researchers:
- Professor Tadashi Yamamoto, OIST
- Professor Amy Shen, OIST
- Mr. Paul Tsai, OIST
3. Activities and Findings
3.1 Inertial and inertioelastic instabilities in microfluidics
3.1.1 Inertioelastic Flow instability at a stagnation point
Noa Burshtein, Konstantinos Zografos, Amy Q. Shen, Robert J. Poole and Simon J. Haward
Published in Physical Review X, November 17, 2017
A number of important industrial applications exploit the ability of small quantities of high molecular weight polymer to suppress instabilities that arise in the equivalent flow of Newtonian fluids, a particular example being turbulent drag reduction. However, it can be extremely difficult to probe exactly how the polymer acts to, e.g., modify the streamwise near-wall eddies in a fully turbulent flow. Using a novel cross-slot flow configuration, we exploit a flow instability in order to create and study a single steady-state streamwise vortex. By quantitative experiment, we show how the addition of small quantities (parts per million) of a flexible polymer to a Newtonian solvent dramatically affects both the onset conditions for this instability and the subsequent growth of the axial vorticity. Complementary numerical simulations with a finitely extensible nonlinear elastic dumbbell model show that these modifications are due to the growth of polymeric stress within specific regions of the flow domain. Our data fill a significant gap in the literature between the previously reported purely inertial and purely elastic flow regimes and provide a link between the two by showing how the instability mode is transformed as the fluid elasticity is varied. Our results and novel methods are relevant to understanding the mechanisms underlying industrial uses of weakly elastic fluids and also to understanding inertioelastic instabilities in more confined flows through channels with intersections and stagnation points.
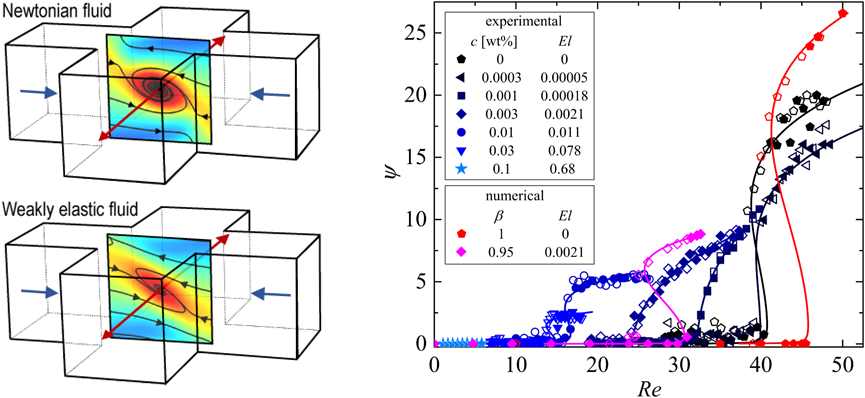
Figure: (Left) The cross-slot geometry where fluid is introduced from two opposite directions (blue arrows) and meet at the center of the geometry around a stagnation point. A special flow configuration allows to obtain quantitative micro-particle imaging velocimetry measurements and reveal the structure of a vortex with a simple Newtonian fluid such as water (top image) and how this vortex changes its structure and intensity with a slight addition of polymer solution (bottom image). (Right) vortex intensity ψ plotted with the Reynolds number (Re) for several fluids with increasing elasticity (El). As El is increased critical Re and vortex intensity decreases. Hysteresis is not consistent in all fluids. Experimental results are in good agreement with numerical simulations.
3.1.2 Elastic modifications of an inertial instability in a 3D cross-slot Numerical study
Konstantinos Zografos, Noa Burshtein, Amy Q. Shen, Simon J. Haward and Robert J. Poole
Submitted to the Journal of Non-Newtonian Fluid Mechanics, 2017
We numerically investigate inertial flows of viscoelastic fluids within a three-dimensional cross-slot geometry of a square cross-section. Our study focuses on the inertial instability that occurs above a critical Reynolds number resulting in a transition to a steady-flow asymmetry. We investigate numerically the effects of elasticity upon its characteristics by employing the upper-convected Maxwell (UCM), the Oldroyd-B and the modified Chilcott-Rallison finitely extensible nonlinear elastic (FENE-MCR) models. In so doing, we show that the UCM and the Oldroyd-B model results are restricted to very low nominal Weissenberg numbers at non-negligible Reynolds numbers, due to a significant increase in the strain-rate at the stagnation point caused by inertia. The resulting steady-asymmetric flow at these critical conditions gives rise to the formation of a single axially-aligned spiral vortex, which is formed along the outlet channels of the geometry in good agreement with experimental observations. Below these critical conditions the flow remains steady-symmetric, varying from a nearly two-dimensional flow at very low Re to a more complex three dimensional flow at higher Re. In a recent publication [Burshtein et al. [Phys. Rev. X, 2017], we demonstrated experimentally, accompanied by limited complementary numerical simulations, the impact of elasticity upon the critical conditions for which the instability develops and the behavior of the subsequent growth of vorticity of the single spiral vortex. Our results here show how different viscoelastic models influence the instability in a 3D cross-slot and demonstrate an interesting behavior of the first normal-stress difference, providing an additional insight on the potential mechanisms which are responsible for the suppression of the spiral-vortex. We also elucidate the role played by solvent-to-total viscosity ratio and the extensibility parameter in the FENE-MCR model on the instability.
Figure: (Left) Weissenberg number (Wi) and (right) effective Weissenberg number (Wieff) as a function of critical Re for all viscoelastic fluids considered in the study. Closed symbols correspond to cases where elasticity is maintained constant while open symbols refer to the cases of constant Weissenberg number. An increase in the polymer concentration (β) causes the transition to be shifted to lower Re when compared to a Newtonian fluid.
3.1.3 Poisseuille Flow Over a Wavy Surface
Simon J. Haward, Jacob Page, Tamer A. Zaki, Amy Q. Shen
Accepted for publication in Physical Review Fluids
We present a detailed series of experiments using spatially-resolved flow velocimetry to examine the flow of Newtonian fluids through rectangular channels with one wavy surface of wavenumber k. The glass channels are fabricated by the novel method of selective laser-induced etching, which allows them to be made with a high (quasi-2D) aspect ratio (width/depth, w/2d = 5) and with an accurate wave profile of small relative amplitude (A/d = 0.05, A < k). Following the prior theoretical work for plane Couette flow over a wavy surface [F. C. Charru and E. J. Hinch, 'Phase diagram’ of interfacial instabilities in a two-layer Couette flow and mechanism of the long-wave instability,” J. Fluid Mech. 414, 195-223 (2000)], we examine the influence of two dimensionless parameters (the normalized channel depth α = kd and the viscous length scale θ) on the penetration depth P of the perturbations that the wavy surface induces to the Poisseuille base flow. The asymptotic analysis by Charru and Hinch predicted three regimes of behavior classified as ‘shallow viscous’ (P ≈ α), ‘deep viscous’ (P ≈ π), and ‘inviscid’ (P ~ θ). All three regimes are here verified experimentally. Minor differences in details of the ‘phase diagrams’ for the ow regimes in α-θ parameter space observed between Poisseuille and plane Couette flow are attributed to the contrasting boundary conditions in the different flow configurations. The experimental results presented here also compare favorably to results from linear theory for a Poiseuille base flow, and thus establish a detailed experimental complement to the theory.
3.1.4 Nonlocal Effects in Viscoelastic Poisseuille Flow Over a Wavy Surface
Simon J. Haward, Jacob Page, Tamer A. Zaki, Amy Q. Shen, In preparation for submission
We present the first experimental evidence to support the recent prediction of nonlocal vorticity amplification by viscoelastic fluids flowing near wavy surfaces. Spatially-resolved flow velocimetry is carried out in rectilinear channels (depth 2d) having one wavy surface (wavelength λ << d, amplitude A << d). Measurements made using a weakly viscoelastic dilute solution of a high molecular weight flexible polymer reveal significant differences compared to the equivalent flow of a Newtonian fluid. The greatest difference is found a significant distance from the wavy surface, and is shown by comparison with linear perturbation results using a common viscoelastic constitutive model to be consistent with a nonlocal vorticity amplification mechanism driven by the polymer torque.
3.1.5 Steady Viscoelastic Flow Around High-Aspect-Ratio, Low-Blockage-Ratio Microfluidic Cylinders
Simon J. Haward, Amy Q. Shen, In preparation for submission
We employ a state-of-the-art microfabrication technique (selective laser-induced etching, SLE) to produce microfluidic cylinder geometries that explore new geometrical regimes. Using SLE, two microchannels are fabricated in monolithic fused silica substrate with height h = 2 mm and width w = 0.4 mm (aspect ratio AR = h/w = 5) containing cylinders of radius r = 0.02 mm (blockage ratio β = 2r/w = 0.1), centered at the channel mid-width, w/2. An ‘sc’ channel contains a single cylinder, while a ‘dc’ channel contains two axially-aligned cylinders separated by a distance L = 1 mm (L = 50r). Compared with cylinder geometries fabricated by soft lithography (which typically have AR << 1 and β ≥ 0.5), these rigid glass devices provide a quasi-2D flow along the direction of the cylinder axis and also more clearly reveal the effects of the strong extensional wake at the trailing stagnation point. Using flow velocimetry and quantitative birefringence measurement techniques we study the flow behavior of a well-characterized viscoelastic polymer solution around the cylinders. The small cylinder radii result in low inertia and very high elasticity numbers El = 2400. For the ‘sc’ device, we report strong flow modification effects around the cylinder as the flow rate is incremented, which we associate with the deformation of polymer molecules in the wake region. Purely elastic asymmetries arise in the flow upstream of the cylinder and the growth of an extremely long downstream wake (up to > 300r) is observed. In the ‘dc’ channel, at lower flow rates equal wakes develop downstream of both cylinders. However, as the wake of the upstream cylinder extends up to L > 50r, the second downstream cylinder becomes encapsulated by the wake of the upstream cylinder and is effectively obviated from the flow field. The results will be of relevance to understanding practical applications of viscoelastic fluids, for example in porous media flows, and also for benchmarking against numerical simulations using viscoelastic constitutive models.
3.2 Microrheometry for dilute polymer solutions
3.2.1 Relaxation time of dilute polymer solutions: a microfluidic approach
Francesco Del Giudice, Simon J. Haward and Amy Q. Shen
Journal of Rheology vo.61 (2) pp. 327-337, 2017 (Cover Image)
Polymer solutions are considered dilute when polymer chains in a solution do not interact with each other. One important step in the characterization of these systems is the measurement of their longest relaxation times λ. For dilute polymer solutions in low-viscous solvents, this measurement can be very challenging through conventional techniques. Recently, several microfluidic platforms have been successfully employed to measure the rheological properties of weakly viscoelastic solutions. Nevertheless, a comparison between data generated from different microfluidic platforms has not yet been presented. In this work, we measure λ of dilute polymer solutions for concentrations down to a few parts per million, by using two distinct microfluidic platforms with shear and extensional flow configurations. We consider three representative polymer classes: neutral polymers in near-theta and good solvents, and a biological polyelectrolyte in a good solvent in the presence of salt. Relaxation times in shear flow λshear are measured through the μ-rheometer based on the viscoelastic alignment of particles in a straight microchannel. Relaxation times in extensional flow λext are measured in a microfluidic optimized cross-slot configuration based on the onset of the flow-induced birefringence. A good agreement between experimental measurements from the two platforms is found. Experimental measures are also compared with available theories.
Figure: a) Comparison between experimental data derived from x-slot measurements and the μ-rheometer for a-polystyrene in DOP (theta solvent) and for a-polystyrene in TCP (good solvent).
3.2.2 When Microrheology, Bulk Rheology, and Microfluidics Meet: Broadband Rheology of Hydroxyethyl Cellulose Water Solutions
Francesco Del Giudice, Manlio Tassieri, Claude Oelschlaeger & Amy Q. Shen
Macromolecules, vol.50(7) pp.2951-2963, 2017
In this work, we present new insights related to a debate on the morphological structure of hydroxyethyl cellulose (HEC) molecules when dissolved in water, i.e., whether HEC adopts a linear-flexible or a rod-like fibrillar configuration. We have employed “seven” rheological techniques to explore the viscoelastic properties of HEC solutions at different time and length scales. This work demonstrates an excellent convergence between various rheological techniques over a broad range of frequencies and concentrations, allowing us to derive microstructural information for aqueous HEC solutions without the use of complex optical imaging techniques. We find that when dissolved in water unmodified HEC behaves like a linear uncharged polymer, with an entangled mass concentration of ce = 0.3 wt%. Moreover, for the first time we provide the concentration scaling laws (across ce) for the longest relaxation time λ of HEC solutions, obtained from direct readings and not inferred from fitting procedures of fluids shear flow curves.
Figure: a) Comparison between the HEC solutions’ linear viscoelastic moduli versus frequency measured with conventional rotational rheometer (BR), with optical tweezers (OT), Diffusive wave spectroscopy (DWS), Dynamic light scattering (DLS) and multiple particle tracking (MPT), for HEC solutions having concentrations of (a) 0.8, (b) 0.5, and (c) 0.4 wt%. The lines are guides for the theoretical predictions. Notice that μ = 1.76 for all the concentrations investigated.
3.3 Shear Rheology of Graphene Oxide Dispersions
Francesco Del Giudice & Amy Q. Shen
Current Opinion in chemical Engineering, vol.16 pp. 23-30, 2017 (Invited Review)
Graphene oxide (G-O) is a chemically oxidized sheet of graphene. Due to their functional groups, G-O sheets have found numerous applications in biomedical, electrical and material engineering. The flow properties of G-O dispersions, i.e., the rheology, are intimately related to the material processing and design of G-O based composites. In this review, we report recent findings on the shear rheology of both aqueous G-O dispersions and composite materials containing G-O sheets. We focus on aqueous G-O dispersions to understand interactions between G-O sheets. Applications related to materials science and technology are also presented.
Figure: a) G-O aqueous dispersions at different volume concentrations are prepared by adding G-O in water. Oscillatory linear shear flow: When the concentration is less than the critical volume or mass concentration, viscous response due to the drag of the G-O sheets prevails over the elastic response. When the concentration is greater than the critical volume concentration, elastic response due to the G-O self-aggregation prevails over the viscous response due to the drag of the clusters. Steady shear flow: When the concentration is less than the critical volume concentration, and at Peclet number Pe<1, G-O sheets are randomly oriented; while at Pe > 1 G-O sheets are oriented along the flow direction. When the concentration is greater than the critical volume concentration and at Peclet number Pe < 1, G-O are arranged in randomly oriented clusters; while at Pe > 1 clusters are broken down. Transient shear flow: Only for the concentration greater than the critical volume concentration is considered. Initially, the dispersion is arranged in randomly oriented clusters. After applying a flow at Pe > 1, clusters are broken down and G-O sheets are oriented along the flow direction. When the flow is arrested, Pe = 0, G-O sheets start to self-arrange. After sufficient resting time, G-O sheets recover the initial cluster configuration.
3.4 Biosensing, Micro/nanopatterning, and Bio-MEMs
3.4.1 Microcontact printing with aminosilanes: creating biomolecule micro-and nanoarrays for multiplexed microfluidic bioassays
S. Sathish, S. G. Ricoult, K. Toda-Peters, and A. Q. Shen
Analyst, 142(10), 1772-1781, 2017.
Microfluidic systems integrated with protein and DNA micro- and nanoarrays have been the most sought-after technologies to satisfy the growing demand for high-throughput disease diagnostics. As the sensitivity of these systems relies on the bio-functionalities of the patterned recognition biomolecules, the primary concern has been to develop simple technologies that enable biomolecule immobilization within microfluidic devices whilst preserving bio-functionalities. To address this concern, we introduce a two-step patterning approach to create micro- and nanoarrays of biomolecules within microfluidic devices. First, we introduce a simple aqueous based microcontact printing (μCP) method to pattern arrays of (3-aminopropyl)triethoxysilane (APTES) on glass substrates, with feature sizes ranging from a few hundred microns down to 200 nm (for the first time). Next, these substrates are integrated with microfluidic channels to then covalently couple DNA aptamers and antibodies with the micro- and nanopatterned APTES. As these biomolecules are covalently tethered to the device substrates, the resulting bonds enable them to withstand the high shear stresses originating from the flow in these devices. We further demonstrated the flexibility of this technique, by immobilizing multiple proteins onto these APTES-patterned substrates using liquid-dispensing robots to create multiple microarrays. Next, to validate the functionalities of these microfluidic biomolecule microarrays, we perform (i) aptamer-based sandwich immunoassays to detect human interleukin 6 (IL6); and (ii) antibody-based sandwich immunoassays to detect human c-reactive protein (hCRP) with the limit of detection at 5 nM, a level below the range required for clinical screening. In summary, with a single printing step, this aminosilane patterning technique enables the creation of functional microfluidic micro- and nano-biomolecule arrays, laying the foundation for high-throughput multiplexed bioassays.
Figure: Multiplexed protein microarrays on APTESaq micropatterns using liquid dispensing robots and microfluidic devices. (a) Schematic illustrating a liquid dispensing robot delivering two different protein solutions to different portions of an APTESaq patterned substrate. (b) Fluorescence images depicting green and red IgGs delivered to different regions of an APTESaq array using a dispensing robot. (c) Schematic of microcontact printed array of 100 μm wide APTESaq stripes within a microfluidic device containing channel arrays aligned perpendicular to each other. (d) A microarray of alternating patterns of red and green labeled IgG created within the microfluidic channels. (Scale bars: 2 mm in (b), 300 μm in insets of (b); 300 μm in (d)).
3.4.2 Novel refractive index biosensing of microcontact printed molecules on lithium niobate
Lithium niobate (LiNbO3) is one of the most multifaceted synthetic materials. It is uniaxial, highly birefringent and has a remarkable combination of piezoelectric, optical properties and photorefractive response. These properties of LiNbO3 have been used to develop a number of surface acoustic wave and electro-optical devices for sensing applications. This project demonstrated, for the first time, the use of lithium niobate as a biosensor that detected local refractive index changes triggered by the presence of biomolecules on its surface. The sensitivity of the sensor was found to be 242±16 nm/RIU. As a case study, we immobilized proteins (IgG antibodies) using micro-contact printing to demonstrate sensing capabilities of the device. The validated proof of concept lays a foundation for developing lithium niobate based novel optical biosensors.
Figure: The figure above shows measurement setup developed to acquire optical resonances from lithium niobate. It also shows how easily proteins can be printed on lithium niobate for the use of bio/chemical sensing applications.
3.4.3. Dual-mode refractive index and charge sensing to investigate complex surface chemistry on nanostructures
Nikhil Bhalla, Doojin Lee, Shivani Sathish, A.Q. Shen, Nanoscale, 9, 547-554, 2017.
Gold nanostructures are a highly attractive class of materials with unique electrochemical and optical sensing properties. The integration of gold nanoislands (for instance AuNIs) on semiconductors (such as field effect devices) is a powerful tool for chemical and biological sensing with convenient electrical and optical readouts. In this project we developed a novel dual-mode charge and refractive index sensitive device integrated with nanoplasmonic islands, for the first time, on insulator–semiconductor junctions.
Figure: The above picture shows a nanoisland measuring both mass and charge of the immobilized aptamers.
The developed nano-metal–insulator semiconductor (nMIS) sensor facilitates simultaneous detection of charge and mass changes on the nanoislands due to the binding of biomolecules. The charging of the nanoislands is traced by using the capacitive field-effect electrolyte–metal–insulator–semiconductor structure and the refractive index changes are quantified by measuring the change in the localized surface plasmon resonances of the nanoislands. To demonstrate the performance of our dual-mode sensor we study the effect of oxygen plasma on immobilized biomolecules. As a case study biotinylated aptamers specific to interleukin 6 (IL-6) were chosen to conduct the immunoassay studies. We confirm that the adsorbed aptamers on the nanoislands do not lose their functionality after exposure to low energy oxygen plasma (< 600 J). This finding is critical for the development of ‘ready-to-use’ microfluidic immunoassay platforms (glass–PDMS based) where immobilizing biomolecules on one of the substrates is often required prior to the bonding of glass and PDMS. The dual-mode sensor also casts insights into the active electrostatic tuning of the electrochemical properties of the nanostructures via the molecular adsorption or biological recognition events, in combination with optical sensing and therefore represents a very promising strategy for many label-free biosensing applications. The investigation can be further expanded to study all kinds of molecular binding events that are associated with variations in either the mass or charge change, involving immunoassay probes such as antibodies, aptamers, affimers and somamers.
4. Publications
4.1 Journals
- Franceso Del Giudice, Manlio Tassieri, Claude Oelschlaeger, Amy Q. Shen, When micro-, bulk- and microfluidics-rheology meet: broadband rheology of hydroxyethyl cellulose water solutions, Macromolecules, 2017, 50 (7), 2951-2963, DOI: 10.1021/acs.macromol.6b02727
- Simon J. Haward and Amy Q. Shen, Microfluidic Flows and Confinement of Worm- like Micelles, Book Chapter in Wormlike Micelles: Advances in Systems, Characterisation and Applications, Edited by Cecile A. Dreiss and Yujun Feng, Soft Matter Series No. 6, The Royal Society of Chemistry, 2017, DOI:10.1039/9781782629788.
- Franceso Del Giudice, Simon J. Haward, Amy Q. Shen, Relaxation time of dilute polymer solutions: a microfluidic approach, Journal of Rheology, 2017, 61, 327. dx.doi.org/10.1122/1.4975933
- Doojin Lee, Cifeng Fang, Aniket S. Ravan, G.G. Fuller, A.Q. Shen, Temperature controlled tensiometry using droplet microfluidics, Lab on a Chip, 2017, 17, 717-726. DOI: 10.1039/c6lc01384h
- Nikhil Bhalla, Doojin Lee, Shivani Sathish, A.Q. Shen, Dual-mode mass and charge sensing to investigate complex surface chemistry on nanostructures, Nanoscale, 2017, 9, 547-554, DOI: 10.1039/C6NR07664E.
- Nikhil Bhalla, Shivani Sathish, and Amy Q. Shen, Novel refractive index biosensing of microcontact printed molecules on lithium niobate, Engineering in Medicine and Biology Society (EMBC), IEEE 38th Annual International Conference of IEEE, 2016, DOI: 10.1109/EMBC.2016.7591141
- Y. Zhao, A. Q. Shen, S.J. Haward, Flow of wormlike micellar solutions around con-fined microfluidic cylinders, Soft Matter, 2016, 12, 8666-8681. DOI: 10.1039/C6SM01597B.
- S.J. Haward, G. H. McKinley, A. Q. Shen, Elastic instabilities in planar elongational flow of monodisperse polymer solutions, Scientific Reports, 2016, 6, 33029. DOI:10.1038/srep33029
- J. J. Cardiel, D. Takagi, H. Tsai, A. Q. Shen, Formation and flow behavior of micellar membranes in a T-shaped microchannel, Soft Matter, 2016, 12, 8226-8234. DOI: 10.1039/C6SM01093H.
- H. Tsai, J. Cheng, H. Chang, T. Yamamoto, A.Q. Shen, Uniform electric field stimulation in multi-well plates using 3D CAD polymeric inserts, Scientific Reports, 2016, 6, 26222; DOI: 10.1038/srep26222.
- D.J. Walls, S.J. Haward, A.Q. Shen, G.G. Fuller, Spreading of miscible liquids, Physical Review Fluids, 2016, 1, 013904, DOI: https://doi.org/10.1103/PhysRevFluids.1.013904
- G. Fotouhi, C. Ogier, J.H. Kim, S. Kim, G. Cao, A. Q. Shen, J. Kramlich, J. Chung, Low cost, disposable cable-shaped Al-air battery for portable biosensors, J. Micromech. Microeng., 2016, 26(5), 55011-55018, DOI: https://doi.org/10.1088/0960-1317/26/5/055011
- L. Xu, J. Peng, M. Yan, D. Zhang, A.Q. Shen, Droplet synthesis of silver nanopar- ticles by a microfluidic device, Chemical Engineering and Processing: Process Intensification, 2016, 102, 186-193, DOI: https://doi.org/10.1016/j.cep.2016.01.017
- S.J. Haward, R. J. Poole, M. A. Alves, P. J. Oliveira, N. Goldenfeld, A. Q. Shen, Tricritical spiral vortex instability in cross-slot flow, Physical Review E, 2016, 93, 031101(R), DOI: https://doi.org/10.1103/PhysRevE.93.031101
4.2 Books and other one-time publications
Nothing to report
4.3 Oral and Poster Presentations
- Shen, Amy Q. Flow of wormlike micellar solutions around confined microfluidic cylinders, International Conderence CoMFoS16, Fukuoka, Japan, Oct 23 (2016).
- Shen, Amy Q. Keynote lecture on the strategies for achieving sustainable development goals, STI-Gigaku 2017, Nagaoka, Japan, Jan 5 (2017).
- Shen, Amy Q., Bhalla, Nikhil. Micro/Nanofluidics Based Platforms for Biosensing Applications, Lab-on-a-chip Asia, Singapore, Dec 5 (2016)
- Shen, Amy Q., Bhalla, Nikhil. NANOPLASMONIC SENSORS FOR SENSITIVE DETECTION OF BIOMOLECULES, International Conference on Single Cell Research 2016, Tokyo, Japan, Nov 16 (2016)
- Burshtein, Noa, Haward, Simon J., Shen, Amy Q. Spiral vortex formation in cross-slot flow, ACS Colloids and Surface Science Symposium, Boston, USA, Jun 5-Jun 8 (2016)
- Haward, Simon J., Burshtein, Noa, Poole, Robert J., Oliveira, Paulo J., Alves Manuel A., Shen, Amy Q. Spiral vortex formation in cross-slot flow, American Physical Society Division of Fluid Dynamics (APS-DFD) 69th Annual Meeting, Portland, USA, Nov 20-22 (2016)
- Haward, Simon J., Burshtein, Noa, Shen, Amy Q. Spiral vortex instability in microfluidic cross-slot flow, American Institute of Chemical Engineers (AIChE) Annual Conference, San Francisco, USA, Nov 13-18 (2016)
- Haward, Simon J., McKinley, Gareth H., Shen, Amy Q. Elastic Instabilities in Planar Elongational Flow of Monodisperse Polymer Solutions, ACS Colloids and Surface Science Symposium, Boston, USA, Jun 5-8 (2016)
- Lee, D. J. Development of an integrated microfluidic platform for interfacial dynamics of immiscible liquids, Internatinoal symposium on microchemistry and microsystems, Hong Kong, May 30 (2016)
- Leung, Hei Man Mandy, Shen, Amy Q. Microfluidic Assisted Polyester Nanoparticle Synthesis for Curcumin Delivery, International Symposium on Drug Delivery and Pharmaceutical Sciences: Beyond the History, Kyoto, Japan, Mar 9 (2017)
- Shen, Amy Q. Microfluidic platforms for biomedical applications, OIST Mini Symposium "Science and Technology at the Interface of Bio-Nano-systems: Challenges and Opportunities, Okinawa, Japan, Apr 26 (2016)
- Walls, Dan, Fuller, Gerry, Haward, Simon J., Shen, Amy Q. Pendant drops and liquid jets in miscible environments, American Institute of Chemical Engineers (AIChE) Annual Conference, San Francisco, USA, Nov 13-18 (2016)
- Bhalla, Nikhil, Sathish, Shivani, Shen, Amy Q. Novel refractive index biosensing of microcontact printed molecules on lithium niobate, IEEE-EMBC Conference, Florida, USA, Aug 16-20 (2016)
- Burshtein, Noa, Haward, Simon J., Shen, Amy Q. Effect of fluid elasticity on spiral vortex formation in cross-slot flow, ACS Colloids and Surface Science Symposium, Boston, USA, Jun 5-8 (2016)
- Del Giudice, Francesco, Sathish, Shivani, Shen, Amy Q. Microfluidic-Based Cell Manipulation In Viscoelastic Fluids, The 20th International Conference on Miniaturized Systems for Chemistry and Life Sciences, Dublin, Ireland, Oct 11 (2016)
- Galvin, Casey J., Baye, James, Shen, Amy Q. Characterizing Human Colon Cancer Tumor Spheroids in a Microfluidic Device, 8th International Symposium on Microchemistry and Microsystems, Hong Kong, Jun 30 (2016)
- Haward, Simon J., Shen, Amy Q. Spiral vortex formation at the intersection of two fluid streams, American Physical Society Division of Fluid Dynamics (APS-DFD) 69th Annual Meeting, Portland, USA, Nov 20-22 (2016)
- Haward, Simon J., Zhao, Ya, Shen, Amy Q. Flow of wormlike micellar solutions around confined microfluidic cylinders, ACS Colloids and Surface Science Symposium, Boston, USA, Jun 5-8 (2016)
- Leung, Hei Man Mandy, Shen, Amy Q. Microfluidic Assisted Polyester Nanoparticle Synthesis for Curcumin Delivery, BioEngineering 2017: BioMEMS, 3D-Bioprinting, Tissue Engineering & Synthetic Biology, Boston, USA, Mar 16 (2017)
- Shivani, Sathish, Ricoult, Sebastien G., Toda-Peters, Kazumi, Shen, Amy Q. Micro and Nanopatterned Aminosilanes for Covalent Grafting of Biomolecules in Multiplexed Microfluidic Bioassays, The 20th International Conference on Miniaturized Systems for Chemistry and Life Sciences, Dublin, Ireland, Oct 9-13 (2016)
5. Intellectual Property Rights and Other Specific Achievements
5.1 Versatile fabrication uniform electric field generation devices for cell stimulation in different sizes of circular multi-well culture plates
H.-F. Tsai, and A.Q. Shen
3D POLYMERIC INSERT TO APPLY UNIFORM ELECTRIC FIELD IN CIRCULAR CULTUREWARE (WO2017047098)
Physiological electric fields (EF) are important in numerous tissue development/remodelling processes. Moreover, in vitro physical stimulations including electrical stimulation, shearing, mechanical stretching have been proposed as important tissue training processes in tissue engineering. Previously, we have reported a three-dimensional computer-aided designed (3D CAD) polymeric insert to stimulate a uniform electric field on cells cultured on standard circular multi-plate cultureware. The advantages over conventional rectangular shaped microchannels are higher cell throughput in a single cultureware due to increased effective stimulation area percentage and ease of use.
We have explored different state-of-the-art fabrication processes for different variants of the 3D CAD design for different sizes of circular cultureware. PDMS, thermoplastic, and medical grade titanium devices fabricated by computer numerical control (CNC) milling, molding, and 3D printing are evaluated for cultureware sizes as small as 12-well plate and as big as 100 mm petri dishes. The fabrication processes are established to produce devices in large volume for collaboration and possible commercialization.
Figure: Versatile material and fabrication process for different sizes of cultureware. (A) A PDMS device for 12-well plate molded from a CNC milled aluminium mill visualized with blue dye. (B) A two-piece mating scheme for commercial device fabrication where top ring containing CAD structures are perfectly mate to a simple circular plug (C) A CNC milled PMMA top ring containing the CAD structure. (D) A 3D printed titanium top ring containing the CAD structure.
5.2. Integrated System for Sampling and Processing a Liquid Suspension
K. Toda-Peters, C. Galvin, D. Lee, K. Funakoshi, S. Sathish and A. Q. Shen
(US Patent Application number: 62/509,244)
Many samples intended for analysis are liquid suspensions, such as whole blood or soil samples. Separating the liquid from the solid is an important step in analyzing the liquid phase of the suspension. Existing techniques often require electricity and/or are expensive. Furthermore, on site testing requires a hand-operated system capable of performing all the steps required to obtain a result. Our invention meets such requirements in an integrated, portable, syringe-like device. This system is capable of accepting an unprocessed liquid suspension sample, processing the sample with at least one fluid stored on the device, and then passing the sample through a separation device, such as a filter, and transmitting the filtered liquid for use in additional operations. An example application of this invention is conducting a point-of-care (POC) immunoassay from a whole blood sample.
A microfluidic immunoassay was carried out by either adding a pre-mixed red fluorescent antibody solution to the buffer cartridge, or by placing on the sampling sponge a red fluorescent antibody and mixing it in the syringe. The red fluorescent antibody was captured by a green fluorescent antibody tethered to the surface of a microfluidic device in fluid communication with the syringe system. These results indicate that antibodies can be passed through the invention and captured in a microfluidic immunoassay device.
Figure: (I) Schematic of the syringe-based device. (II) Immunoassay performed within a microfluidic device (a) patterned with green fluorescent antibody to capture (b) complimentary red fluorescent antibody. (c) Depicts overlayed images of (a) and (b).
5.3. Grants and Fellowships
1. Noa Burshtein, Apr 2016 – Mar 2019; JPY 3,600,000; JSPS Research Fellowship (DC1), Japan.
2. Hsieh-Fu Paul Tsai, Apr 2016 – Mar 2019; JPY 3,600,000; JSPS Research Fellowship (DC1), Japan.
3. Mandy Leung, Apr 2016 – Mar 2018; JSPS Postdoctoral Fellowship, Japan.
6. Meetings and Events
6.1 Seminars
1. Prof. Steve Granick (IBS Center for Soft and Living Matter)
- Date: April 20, 2016
- Venue: OIST Campus, C016
- Seminar: Some Surprises and Open Questions in Soft Matter
2. Prof. Dino Di Carlo (UCLA)
- Date: June 30, 2016
- Venue: OIST Campus, C016
- Seminar: Microstructured Materials
3. Prof. Bud Homsy (UCSB, University of Washington)
- Date: July 19, 2016
- Venue: OIST Campus, C209
- Seminar: Interfacial Fluid Mechanics: New Twists on an Old Subject
- Date: July 20, 2016
- Venue: OIST Campus, C209
- Seminar: Greatest Hits of the 60s and 70s: Progress in Fluid Mechanics in the last 5 Decades
4. Prof. Paolo A. Netti (University of Naples Federico II)
- Date: August 31, 2016
- Venue: OIST Campus, C016
- Seminar: Building functional 3D human tissue in vitro: role of the materials and processes
5. Prof. Carlos Rinaldi (University of Florida)
- Date: September 26, 2016
- Venue: OIST Campus, C015
- Seminar: Rotational Dynamics of Nanoparticles in Complex and Biological Fluids
6. Prof. Gerald G. Fuller (Stanford University)
- Date: September 28, 2016
- Venue: OIST Campus, C016
- Seminar: Biofilm-assisted adhesion onto bladder cell
- Date: October 3, 2016
- Venue: OIST Campus, C016
- Seminar: The Role of Asphaltenes in Coalescence in Oil/Water Emulsions
7. Prof. Lok Kumar Shrestha (National Institute for Materials Science (NIMS))
- Date: October 5, 2016
- Venue: OIST Campus, D015
- Seminar: Fullerene Nanoarchitectonics: from Zero to Higher Dimensions
8. Prof. Danny Chung (Chung-Yuan Christian University)
- Date: November 8, 2016
- Venue: OIST Campus, C016
- Seminar: Sensor Interfacing Cicuit Design for Diversified Applications of Electronic Tongue System
9. Prof. Takuya Katashima (Osaka University)
- Date: November 9, 2016
- Venue: OIST Campus, D014
- Seminar: Rheological property of interpenetrating network with permanent and transient networks
10. Dr. Brice Saint-Michel (ENS de Lyon)
- Date: January 10, 2017
- Venue: OIST Campus, C016
- Seminar: Local Rheology using ultrasound echography
11. Prof. Shiro Tsukamoto (National Institute of Technology (NIT), Anan College)
- Date: January 17, 2017
- Venue: OIST Campus, C016
- Seminar: Pd-catalysts on S-terminated GaAs and GaN substrates for green chemistry
12. Dr. Atsushi Matsumoto (Osaka University)
- Date: January 18, 2017
- Venue: OIST Campus, C016
- Seminar: Relationship between Viscoelastic Properties and Chemical Structures of Polymerized Imidazolium-Based Ionic Liquids with Various Counter Anions
13. Dr. Mondher Bouteraa (the University of Lorraine)
- Date: January 23, 2017
- Venue: OIST Campus, C016
- Seminar: Rayleigh-Bénard convection in shear-thinning fluids : Theoretical and experimental approaches
14. Prof. John de Bruyn (University of Western Ontario)
- Date: February 21, 2017
- Venue: OIST Campus, C016
- Seminar: Restricted Diffusion in Soft Matter and Biological Cells
15. Prof. Ronald Larson (University of Michigan)
- Date: February 28, 2017
- Venue: OIST Campus, C016
- Seminar: Multi-Scale Modeling of Semi-flexible Micelles and Polymers
16. Prof. Hajime Tanaka (University of Tokyo)
- Date: March 2, 2017
- Venue: OIST Campus, D014
- Seminar: The structural origin of water’s anomalies
17. Prof. Haruko Takeyama, Prof. Takafumi Inoue, Prof. Toru Asahi, Ms. Eu Ju, Mr. Kosuke Kataoka
- Date: March 7, 2017
- Venue: OIST Campus, C209
- Talk 1: Droplet microfluidics for precise and high thoughput whole genome amplification toward single-cell genome sequencing (Prof. Haruko Takeyama)
- Talk 2: Cytosolic molecular dynamics observed with FCS in neurons (Prof. Takafumi Inoue)
- Talk 3: Toward the molecular mechanism of neuropsychiatric and neurodegenerative disorders (Prof. Toru Asahi, Ms. Eu Ju, Mr. Kosuke Kataoka)
- Seminar information
18. Prof. Ashis Kumar Sen (Indian Institute of Technology, Madras)
- Date: March 15, 2017
- Venue: OIST Campus, D015
- Seminar: Interfacial phenomena in microfluidics
19. Dr. Kazuya Fujimoto (Kyoto University)
- Date: March 22, 2017
- Venue: OIST Campus, D015
- Saminar: Control and measurement of kinesin motility using micro/nano fabricated devices
6.2 Events
2. OIST Workshop "New Aspects of Micro- and Macro-scopic Flows in Soft Matters"
7. Other
Nothing to report.