Membrane Cooperativity Unit (Akihiro Kusumi)
Welcome to the web page of the Membrane Cooperativity Unit, led by Prof. Aki Kusumi.
Overview
We at the Membrane Cooperativity Unit strive to understand how biological membranes work at very fundamental levels by using unique approaches; observing and manipulating each individual single molecule in living cells at a time resolution of 20 µs (1,650x faster than normal video rate). We focus on the plasma membrane among various cellular membranes. The plasma membrane is the outermost cellular membrane which encloses the entire cell. It is critically important for the cell - the fundamental unit of life - because it defines the space for it. The plasma membrane exchanges information, energy, and substances with the outside world, but more interestingly, it functions as a computer to regulate these exchanges, a function generally called “signal transduction.” Our laboratory is dedicated to (1) developing unique methodologies of single-molecule observation-manipulation in living cells (Fig. 1), and (2) elucidating the mechanisms for plasma membrane organization and function, with particular emphases on signal transduction and neuronal network formation, by extensively using single-molecule technologies (Fig. 2).
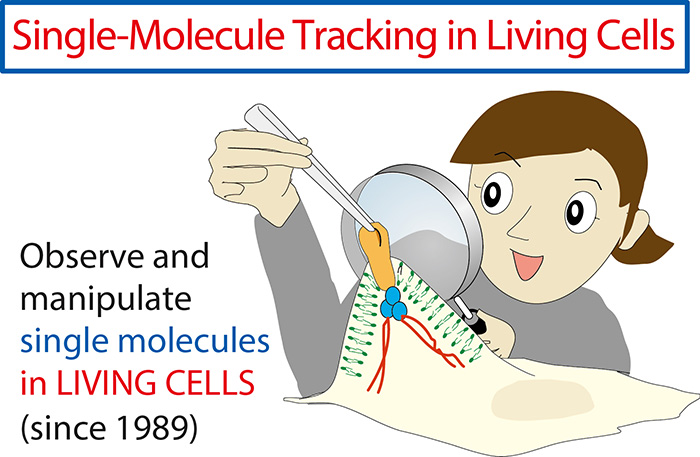
Figure 1 : One of the basic strategies of our laboratory is to observe and manipulate single molecules in living cells by developing new technologies based on a sort of a magnifying glass and molecular chopsticks or laser tweezers.
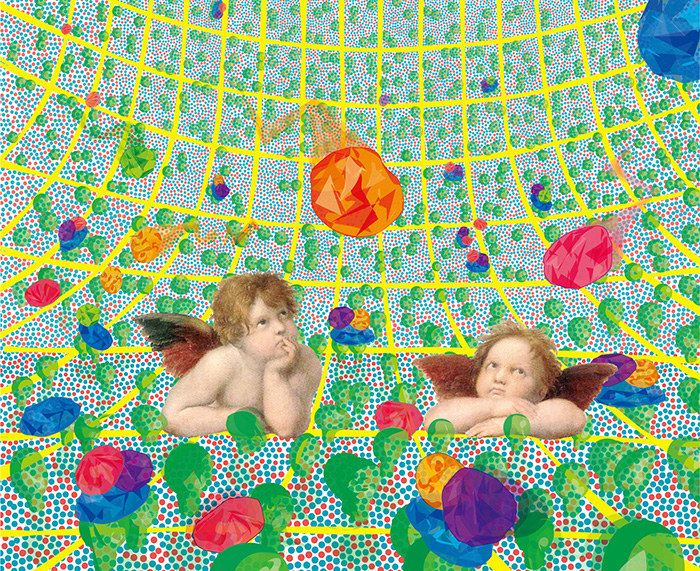
Figure 2 : What the cupids of Raphael’s Madonna Sistina (now in Dresden) quizzically regard might be---how signaling and scaffolding proteins diffuse to arrive at activated receptors in the plasma membrane, one of the key steps for signal transduction. The plasma membrane is partitioned into small compartments by the actin-based membrane skeleton (which the cupids rest on), and its associated transmembrane proteins. Like these cupids, we are observing and manipulating single molecules and molecular interactions.
The plasma membranes of all cells existing on earth have the common structure of a "two-dimensional liquid" (see Fig. 3-(1)). Such universality is comparable to that of the double helical structure of DNA. Like many DNA functions are based on its double helical structure, various plasma membrane functions are enabled by the fluid structure of the plasma membrane. This raises the possibility that we can identify the set of physical properties of the plasma membrane, like those of DNA, that make the plasma membrane work.
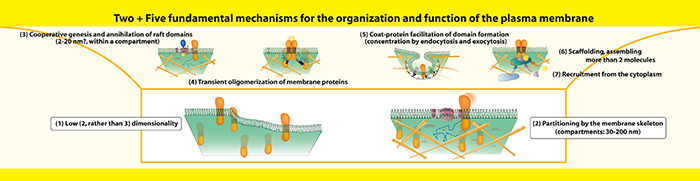
Figure 3 : Two + Five fundamental mechanisms for the organization and function of the plasma membrane. We believe that two of the most fundamental organizing-functioning mechanisms of the plasma membranes are: (1) Low dimensionality (which dramatically enhances molecular collisions in/on the membrane); (2) Partitioning of the entire plasma membrane into 30 to 200 nm compartments by the actin-based membrane skeleton (fence) and various transmembrane proteins anchored to and lining the fence (pickets), which induce a strong hydrodynamic dragging effect around them.
The five additional cooperative regulatory mechanisms we propose are: (3) Metastability, due to various degrees of mutual (im)miscibility of constituent molecules, leading to the cooperative assembly and disassembly of microdomains, including raft domains; (4) Transient oligomerization of membrane proteins; (5) Coat-protein facilitation of domain formation, mainly for endocytosis and exocytosis, varying the local concentrations of certain molecular species within a compartment; (6) Scaffolding, transiently assembling 3 or more molecules; (7) Recruitment from the cytoplasm to the plasma membrane, to take advantage of the 2D surface reaction field in 3D space, where molecules can diffuse even after being adsorbed. These seven (two + five) mechanisms work in concert in the plasma membrane.
Such fundamental mechanisms are likely to be those utilizing the low (two)-dimensionality and modifying the mobility, local concentration, and assembly-dissociation of molecules in-on the plasma membrane. As one of such mechanisms, and perhaps one of the most important mechanisms, we identified the partitioning of the plasma membrane by the meshwork of actin filaments, called actin membrane skeleton (Fig. 3-(2)). In our continued efforts, we found many examples where cooperative assembly of molecules, rather than simple interaction of two molecules, is responsible for key steps in signal transduction and neuronal network formation. Thus, our unit is called “Membrane Cooperativity Unit.” A critically important point here is that many such functional assemblies were transient ones with lifetimes of the orders of submilliseconds to seconds, i.e., they disintegrated right after they formed (and worked), suggesting that the delicate balance between weak cooperativity and thermal fluctuation is important for biological functions (Fig. 4). We now envisage five more cooperative processes are of fundamental importance for the organization and function of the plasma membrane (Fig. 2 (3)~(7)). In particular, we are now trying to understand the three-tiered meso-scale (2~300 nm) domain architecture of the plasma membrane (Fig. 5), which, I think, provides an excellent perspective on the mechanisms of various functions of the plasma membrane.
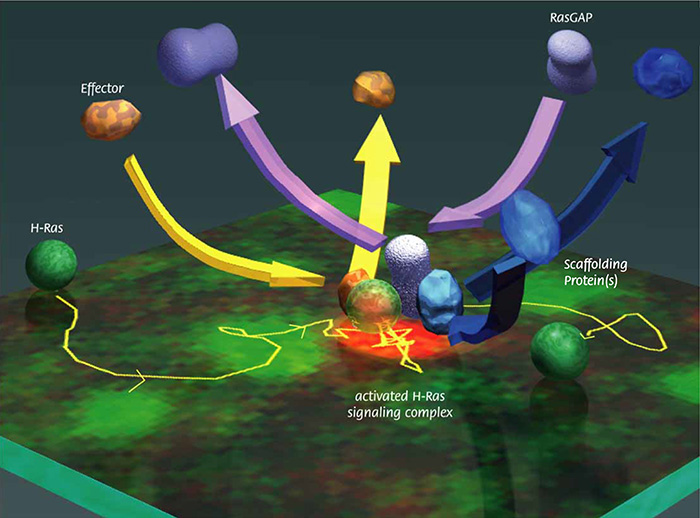
Figure 4 : Artist view of transient, cooperative assembly of activated H-Ras, its effector Raf, and their scaffolding proteins, followed by their dissociation and binding by RasGAP, which deactivates H-Ras, superimposed on the real single-molecule image of H-Ras (the spots in red in the center shows the activated H-Ras) and the trajectory of this H-Ras molecule, on the cytoplasmic surface of the plasma membrane (top surface in this image). This image also shows that we can now observe not only the movement of single molecules but also the activation of single molecules.
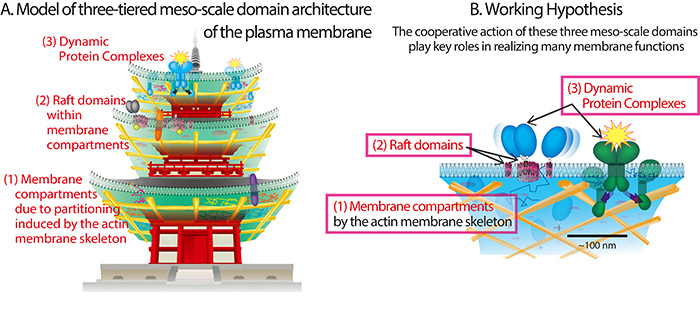
Figure 5 : (A) The concept of three-tiered meso-scale (2~300 nm) domain architecture of the plasma membrane, which, we think, provides an excellent perspective on the mechanisms for various functions of the plasma membrane. The three hierarchically organized domains are the following. The first and most basic tier in this hierarchical architecture is the membrane compartments, formed due to the partitioning of the entire plasma membrane by the actin-based membrane skeleton. The second tier is the raft domain, which are localized within the membrane compartments. The third tier is dynamic protein complexes, with lifetimes of the orders of milliseconds to seconds. (B) Of course, in the real plasma membrane, these three domains coexist in a single membrane and work in concert.
Details of our earlier work can be found at http://www.nanobio.frontier.kyoto-u.ac.jp/index.html.