FY2019 Annual Report
Formation and Regulation of Neuronal Connectivity Research
Visiting Professor David Van Vactor
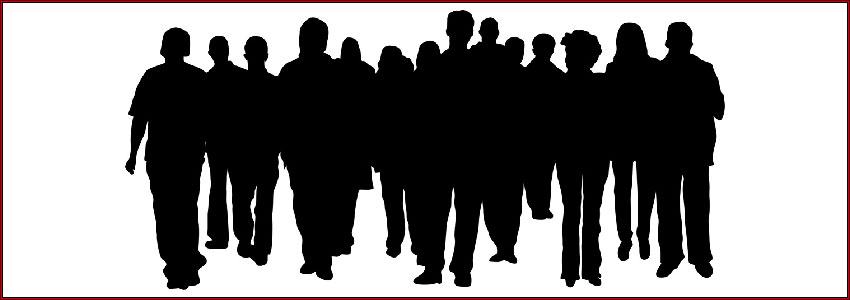
Abstract
The formation, refinement and maintenance of connectivity across complex neural circuits involves multiple levels of regulatory control over gene expression, from the production of mRNA to its processing, transport and translation. Our highly collaborative research program is focused on understanding post-transcriptional mechanisms essential for synaptic development and maintenance, and the networks of effector genes that mediate these key regulatory pathways. Using the genetic model organism Drosophila, we study transcriptome regulation within the motor system, with specific emphasis on the neuromuscular junction (NMJ) that has proven to be a powerful model for analysis of synapse function, development and disease. Our first project (supervised by Group Leader 1) concerns the gene Survival of Motor Neurons (Smn), ortholog of the gene causal to Spinal Muscular Atrophy (SMA), currently thought to mediate synapse development, function and stability and protect motor neurons from degeneration and ultimate death (in collaboration with Drs. Rubin and Gama-Carvalho). Our second project (supervised by Group Leader 2) concerns small, non-coding microRNAs that modulate the stability and translation of mRNAs required for synapse development and plasticity. Through the combined application of transcriptome analysis and functional analysis in this genetic model, we are mapping the conserved mRNA targets of Smn and microRNAs relevant to synapse biology in order to identify key nodes and molecular pathways essential for synapse form and function. Finally, our unit has the responsibility to maintain the Drosophila Genetics Core Facility to make this model organism accessible for all units at OIST. In addition to our research activities, our unit also hopes to maintain existing educational programs such as the Developmental Neurobiology Course (DNC) and the Collaborative Experimental Design and Analytics (CEDA) workshop for undergradautes.
1. Staff
- Dr. David Van Vactor, Professor
- Dr. Takakazu Yokokura, Group Leader (Project 1 & Drosophila Core)
- Dr. Cecilia Lu, Group Leader (Project 2) (- Jul, 2019)
- Ms. Thi Thu Van Dinh, Technician
- Ms. Rika Nakamura, Technician (- Oct, 2019)
- Mr. Akeen Edwards, Research Assistant
- Ms. Chie Edwards, Research Unit Administrator
2. Collaborations
2.1 Theme: Computational analysis of RNA splicing patterns in high-content RNAseq datasets, and conservation of RNA splicing and stability effects from Drosophila to human models of Spinal Muscular Atrophy (SMA).
- Type of collaboration: Joint research
- Researchers/Team Member:
- Dr. Margarida Gama-Carvalho (University of Lisbon, Lisbon, Portugal)
- Professor David Van Vactor
- Dr. Takakazu Yokokura
- Seiko Yoshikawa
- Thi Thu Van Dinh
- Kun Xiao
2.2 Theme: Defining patterns of RNA splicing and stability characteristic of human IPS-derived mixed motor neuron model of Spinal Muscular Atrophy (SMA).
- Type of collaboration: Joint research
- Researchers:
- Dr. Lee Rubin (Department of Stem Cell and Regenerative Biology, Harvard University, Cambridge, MA, USA)
- Professor David Van Vactor
- Dr. Takakazu Yokokura
- Seiko Yoshikawa
3. Activities and Findings
3.1 Spinal Muscular Atrophy (SMA)
1) Generation of Drosophila Smn Transcriptome Data
Due to the functional impact of SMA on neurons and synapses in both vertebrate and invertebrate species, we chose to focus our initial transcriptome analysis on neural patterns of gene expression. Our previous analysis of Smn alleles in Drosophila revealed that tissue-specific RNA interference (RNAi) provides an effective dose-dependent mimic of human SMA that avoids early the lethality and pleiotropy which interfere with analysis of mature neuromuscular synapses [2, 43]. In order to select a genotype in which Smn expression levels in CNS are at comparable levels of Smn expression in SMA patients, we reduced Smn in nervous system using (P{UAS-SmnRNAiC24}) driven by pan-neural driver elav (P{GAL4-elav.L}1) in otherwise wildtype background (C24) or in the presence of heterozygous Smn deletion (Df(3L)SmnX7). Smn expression levels in CNS dissected from the third instar larvae were examined by quantitative RT-PCR (qRT-PCR). Smn RNAi (C24) in a wildtype background reduced expression levels of Smn in CNS only 20% compared to genetically matched wild type controls (PWIZ). In contrast, Smn levels in CNS were decreased to 40% of wildtype levels in the X7C24 background similar to SMN levels in patients with type 2 and type 3 SMA. Comparable results were obtained using primer combinations targeting the C-terminal or N-terminal half of Smn gene. Thus, we selected X7C24 as the Smn mutant for our transcriptome analysis.
Although cell sorting technology has been used often to profile gene expression, dissociation of the CNS severs neurites and induces acute stress that can significantly alter mRNA levels. Thus, we decided to use gentle dissection of whole larval CNS as source for our tissue material for total RNA sample preparation and the subsequent RNA-seq pipeline. Each sample consisted of 200 ventral nerve cords dissected from crawling third instar larvae; other tissues such as imaginal discs, fat body, salivary glands and gut were carefully removed. From our recent work with this protocol [40], we developed a rapid means for quality control (QC) using qRT-PCR with a panel of ten sentinel gene markers to identify and eliminate samples with contamination from non-neuronal tissues. After RNA samples passed this initial QC step, each biological replicate was monitored for optimal RNA fragmentation, cDNA synthesis and adapter ligation to minimize variability that might confound quantitative comparisons between RNA-seq datasets. Once cDNA libraries were prepared for each replicate, digital PCR was used to estimate library complexity before amplification. Careful optimization of library amplification revealed that although 15 cycles of PCR amplification of a cDNA library is a standard protocol for generating a sequence library, 40 to 70% of reads in such samples that were aligned to genome represented duplication artificially generated by PCR artefacts [40]. Optimization showed that clone numbers contained in sequence libraries were exponentially increased up to 10 PCR cycles starting with cDNA libraries containing approximately 1.3x109 clones, whereas amplification was saturated above 10 cycles. Thus, we used 1.0x109 cDNA as template and run 10 cycles of PCR reaction to generate each library. Sequence-tagged primers were used to allow libraries to be combined during sequencing as previously described. After libraries were generated, we examined the copy number of each library by running digital PCR and the size distribution of library by using a BioAnalyzer2100 apparatus. If a sequence library contained more than 2x1012 clones, or the majority of clones was over 600-bp, we excluded it from following RNA-seq analysis. The libraries that passed this set of criteria were then submitted for paired-end sequencing with a 100 bp average read length on an Illumina HiSeq2000 apparatus.
2) Initial Analysis of Drosophila Transcriptome
Once raw Drosophila RNA-seq data of sufficient read-depth was obtained from the larval CNS libraries, sequences were pre-processed to remove low quality reads using FastQC prior to mapping and alignment. Clustering analysis showed that the biological replicates were highly correlated. After removal of duplicate sequences, the mapped dataset was further processed and normalized in order to perform quantitative comparisons between Smn and wild type samples. Differential expression (DE) was assessed using the DESeq algorithm, whereas DEXSeq was utilized to generate exon counts in order to identify genes with Differential Exon Usage (DEU). Statistical analysis of the DE datasets from experimental and control revealed that 1,397 genes displayed significant (FDR < 0.05) deviation from their wild type counterparts in the Smn mutant libraries. The fold-change values varied across a large range for DE genes. Concomitantly, 1,354 DEU hits were found, however, the mean difference in isoform levels between Smn and control were much lower. When the DE and DEU gene sets were compared, we found that more than 25% of the DE genes also represented DEU changes and vice-versa.
Previous analysis of systemic mutations showed that complete loss of Drosophila Smn induces a significant developmental arrest associated with an elevated stress response connected to the JNK pathway. Although our Smn RNAi (X7C24) model of SMA in Drosophila does not show overt signs of developmental arrest at mid-larval stages, we looked for evidence of significant developmental delay in gene expression that might bias our analysis of Smn-dependent genes and pathways. Using available RNA-seq expression data for L1, L2 as well as mid and late L3 larval stages available on FlyBase, it was possible to define gene expression markers characteristic of early (56 genes) or late (7 genes) larval instars. If our X7C24 animals were delayed, we would anticipate an enrichment of early markers among the genes we detected to be Smn-dependent, however, we found that only 4 early-specific genes were part of the transcriptome alterations detected in our study. Thus, we conclude that our SMA model does not induce a significant developmental delay
3) Validation of DE and DEU genes
Although we prepared RNA-seq libraries carefully to limit biological variability and reduce artefactual sequence data, it was important to perform an independent validation of our findings. Thus, we selected a subset of DE and DEU genes for detection of steady state expression changes in gene expression using qRT-PCR on independently collected RNA samples. First, we tested ten DE genes with various levels of expression in wildtype sequence data; primers were designed for the qRT-PCR assay by targeting regions commonly used among all splice isoforms. Eight out of ten DE genes exhibited the same directionality of changes in expression in both RNA-seq and qRT-PCR (Pearson’s correlation coefficient R2 = 0.838). Next, we examined genes that displayed significant DEU patterns in the RNA-seq dataset. We randomly selected genes reportedly expressed in the third instar larval CNS [http://FlyBase.org/] across a range of expression levels for validation. In order to select candidate exons of that expression were above our qRT-PCR detection threshold, we set two criteria: (i) that candidate exons displayed 100 or more RNA-seq reads, and (ii) that qPCR primers and probes were unique to the gene and represented the candidate exon. Overall, 25 exons in 19 DEU genes were selected for validation, and 21 exons showed the positive correlation in the directionality of fold changes between RNA-seq and qRT-PCR assay (correlation coefficient R2 = 0.814). Not surprisingly, we found the RNA-seq analysis to be more sensitive; exons with absolute fold changes less than 0.6 in our RNA-seq data tended to show fold changes by qRT-PCR assay that were less significant.
4) Defining a Conserved Population of Smn-dependent Genes
Although the Drosophila model for SMA displays many of the phenotypic hallmarks of the human disease, arguing for conservation of SMN function and the value of our various disease models [2, 42, 43], we wanted to explicitly compare SMN-dependent transcriptome data across species to ask if the broader regulatory impact of SMA might be conserved from fly to human. Because the Drosophila larval CNS represents a mixture of neuronal identities, we needed a mixed population that would include motor neurons but not be exclusive to them. Thus, to generate RNA samples from neuronal cell types derived from human SMA patient genotypes (from Dr. Lee Rubin), we used iPS cells from patient fibroblasts that had been differentiated in vitro to generate mixed neuronal cultures rich in motor neurons. We processed samples from three classes of SMA patients (type I, II and III) using a workflow comparable to that used for the fly samples at the library preparation and sequence analysis steps.
The sequencing of the human samples generated excellent read-depth and overall quality. To validate the samples, we quantified the abundance of SMN transcripts using the RNA-seq data and independent qRT-PCR and found the expected relative abundance of SMN1 and SMN2 RNA. Overall, of the 19,825 genes were detected, 7,963 genes were either positively or negatively correlated with SMN2 levels. Principle component analysis (PCA) also showed that biological replicate data from each SMA patient was tightly clustered; however, unlike the Drosophila system where genetic background differences between experimental and control can be minimized, isogenic controls were not available for the three human SMA genotypes (SMA I-III). Thus, for a conservative identification of SMN-dependent genes, we focused only on genes (for DE) or exons (for DEU) that were found to be differentially expressed and that changed in the same direction in all three human genotypes (we operationally defined this overlap as “core” human SMA). Within this overlap between human SMA I-III, we identified 2,192 human DE genes and 820 human DEU genes. The overlap between human DE and DEU was relatively small, analogous to our Drosophila RNA-Seq results.
To assess conservation across species, dipteran orthologs for each of these 2,891 ‘core’ human SMA genes were identified using the DRSC integrative ortholog prediction tool (DIOPT), and an overlap with the genes from our current Drosophila SMA RNA-seq dataset was identified. A similar approach was used to identify human orthologs of the previously defined DE and DEU Drosophila gene sets; the reverse comparison was also made because gene family size and annotation influences definition of orthologs. Overall, after merging the lists of orthologs, a highly significant percentage of the significant hits in Drosophila were conserved across species (573 Dme genes versus 594 Hsa genes). The fact that the overlap between fly hits and human orthologs represented 24% was highly encouraging because only ~61% of the Drosophila genome is conserved to human overall.
5) Analysis of Muscle Transcriptome using the Drosophila SMA Model
In 2019, we began a parallel project to obtain and analyze transcriptome data from the same genotyeps that we used for the CNS transcriptome of the Drosophila SMA Model. Although SMA is known to effect muscle tissue, the downstream pathways relevant to muscle in our system have not been mapped.
6) Conclusions
The Survival of Motor Neuron (SMN) gene family encodes a set of highly conserved proteins thought to perform comparable functions in diverse organisms from ecdysozoa to vertebrata. For example, these small RNA-binding proteins interact with a common core of partners, including the Gemin proteins required for the assembly of small nuclear ribonuclear protein (snRNP) complexes that play an important role in nuclear RNA processing. Indeed, reduction of SMN activity in metazoan species as distinct as fruit flies, roundworms, zebrafish, mice and humans leads to strikingly similar phenotypic effects on motor circuits and neuromuscular synapses characteristic of the disease Spinal Muscular Atrophy (SMA), suggesting that SMN-dependent mechanisms in the nervous system converge on a set of common pathways. However, because SMN proteins display multiple functional properties, multiple protein-protein interactions, and multiple modes of localization in vivo, it has been challenging to discern the relevant set of genes that depend on SMN activity for the maintenance of normal motor circuit function. For these reasons, we undertook an analysis to define conserved and neurally-expressed genes that display SMN-dependence in both Drosophila and human. Our results show that the overlap in nearly six hundred potential targets of SMN function is highly significant across species, reinforcing the value of invertebrate genetic models to probe the molecular mechanisms underlying SMN phenotypes in the nervous system.
4. Publications
4.1 Journals
Nothing to report
4.2 Books and other one-time publications
Nothing to report
4.3 Oral and Poster Presentations
- Yokokura, T., McNeill, E., Yoshikawa, S., Dinh, T.T.V., Van Vactor, D. Defining function of canonical pathway in SMA motor neuron and NMJ pathology; Anaheim, CA. June 27-30, 2019
- Yokokura, T., McNeill, E., Yoshikawa, S., Dinh, T.T.V., Xiao, K., Nakamura, R.,Van Vactor, D. Defining function of canonical pathways in SMN dependent motor neuron abnormality and NMJ pathology; Cold Spring Harbor, NY, Oct. 1-5, 2019
- Yokokura, T., McNeill, E., Yoshikawa, S., Dinh, T.T.V., Van Vactor, D. Defining function of canonical pathways in SMN dependent neuromuscular pathology; Evry, France. Feb 5-7, 2020
- Van Vactor, D., McNeill, E., Yoshikawa, S., Dinh, T.T.V., Yokokura, T. Modeling SMA in Drosophila (Keynote lecture): Annual Symposium, Developmental Biology Training Program, University of Pennsylvania, Philadelphia, PA.
- Van Vactor, D., McNeill, E., Yoshikawa, S., Dinh, T.T.V., Yokokura, T. Modeling SMA in Drosophila (Seminar): HMS Initiative for RNA Medicine Seminar Series, Beth Israel Deaconess Medical Center Cancer Center, Boston, MA.
- Van Vactor, D., McNeill, E., Yoshikawa, S., Dinh, T.T.V., Yokokura, T. Modeling SMA in Drosophila (Seminar): Department of Biochemistry & Cellular and Molecular Biology, University of Tennessee, Knoxville, TN.
5. Intellectual Property Rights and Other Specific Achievements
5.1 Development of research equipment with the Drosophila Genetics Core
We have initiated to develop a system to record and analyze behavior of adult Drosophila with JRC Co. Ltd. (Osaka city, Osaka 550-0011, Japan).
6. Meetings and Events
6.1 Developing Neural Circuits Course (former Developmental Neurobiology Course)
- Date: Jul. 29 ~ Aug. 12, 2019
- Venue: OIST Campus
- Co-organizers: Drs. Ichiro Masai, Hiroshi Kawasaki, Hiroshi Kohsaka, David Van Vactor, and Yoko Yazaki-Sugiyama
- Lecturers: Yang Dan (UC Berkeley), Filippo Del Bene (INSERM), Wen-Biao Gan (NYU), Paul Garrity (Brandeis University), Jesse Goldberg (Cornell University), Stephanie Gupton (UNC), Joshua Johansen (RIKEN CBS), Ikue Mori (Nagoya University), Akinao Nose (University of Tokyo), Suzanne Paradis (Brandeis University), Naoshige Uchida (Harvard University), Kristin White (Harvard University), Yutaka Yoshida (OIST/Cornell), Rafael Yuste (Columbia University), Michisuke Yuzaki (Keio University)
6.2 Collaborative Experimental Design and Analytics Workshop 2020
The Collaborative Experimental Design and Analytics 2020 is designed to present students with thematically related research topics in the biological sciences, from organismal to molecular resolution, which represent phenomena that illustrate fundamental principles by combining scientific research presentations, foundational lectures, hands-on data collection and quantitative skills workshops. This one-week intensive course will provide opportunities for students to explore cutting-edge questions in a beautiful campus at Okinawa Institute of Science and Technology Graduate University (OIST), Japan.
- Title: Quantitative Analysis of Behavior & Neurobiology
- Date: March 1-10, 2020 (CANCELLED due to COVID 19 pandemic)
- Venue: OIST Campus Lab1
- Co-organizers: Drs. David Van Vactor , Ichiro Maruyama (OIST), Elizabeth McNeill (Iowa State University) , and Hiroshi Kohsaka (the University of Tokyo)
7. Other
7.1 Drosophila Genetics Core Facility
1) Mission
Drosophila melanogaster has been widely used as one of model organisms in biological and medical research field, because this system offers a rich and sophisticated set of genetic tools and reagents for analysis of complicated biological events in relatively simple context. As a state-of-the-art research institution, the Okinawa Institute of Science and Technology Graduate University (OIST) recognised the value of an accessible facility where anyone in the OIST research community can conduct experiments using Drosophila and enjoy technical support and expert consultation. Our purpose is to support members of OIST research community who consider to use Drosophila as one of their biological research vehicles, and to provide a platform for hands-on educational use of the model to teach a wide variety of biological approaches and concepts to students of multiple stages in career development.
2) Service
Services currently the OIST Drosophila Genetics Core provides: • maintaining essential reagents (Drosophila strains and DNA vectors) • preparing Drosophila culture media • acquiring, setting up and maintaining essential equipment • monitoring the condition of the Drosophila facility • training of research staff (staff scientists, post docs, or technical staff) and students who are not familiar with Drosophila. • Consultation on designing experiments or screens using Drosophila
3) Staff
- Dr. Takakazu Yokokura, Director
- Ms. Thi Thu Van Dinh, Technician
4) Available equipment
- Stereo dissecting microscopes with CO2 pad for genetics (5)
- Stero dissecting microscopes for fluorescent protein expression screening (1)
- Low magnification fluorescence station for live imaging/timelapse/GCAMP
- Image processing and analysis station with Imaris software
- Upright microscope for transmitted lght/ DIC and image acquisition
- Larval locomotion tracking and analysis station
5) Develop equipment
The Core has initiated to develop a system to record and analyze behavior of adult Drosophila with engineers at JRC Co. Ltd.
6) Symposium and Seminar
- Title: Systems for the Study of Neurobiology
- Date: March 6, 2020 (postponed due to COVID-19 pandemic)
- Speaker:
- Dr. Mason Klein (University of Miami)
- Dr. Hiroshi Kohsaka (the University of Tokyo)
- Dr. Ichiro Maruyama (OIST)
- Dr. Elizabeth McNiell (Iowa State University)
- Dr. Takakazu Yokokura, Director
- Dr. Aakanksha Singhvi (Fred Hutchinson Cancer Institute)
7) Publication by Drosophila Genetics Core Users (since established in 2015)
(Note that publications by member of the Formation and Regulation of Neuronal Connectivity Research Unit are not included.)
- Carmen Emborski and Alexander S Mikheyev. Ancestral Diet Transgenerationally Influences Offspring in a Parent-Of-Origin and Sex-Specific Manner. Philos Trans R Soc Lond B Biol Sci. (2019) Mar 18;374(1768):20180181. Doi: 10.1098/rstb.2018.0181
- Zdeněk Lajbner, Reuven Pnini, M. Florencia Camus, Jonathan Miller and Damian K. Dowling. Experimental Evidence That Thermal Selection Shapes Mitochondrial Genome Evolution. Sci Rep. (2018) June 22; 8(1):9500. doi: 10.1038/s41598-018-27805-3.
- Jarosław Bryk, R. Guy Reeves, Floyd A. Reed and Jai A. Denton. Transcriptional effect of a positive feedback circuit in Drosophila melanogaster. BMC Genomics. (2017) Dec.28; 18(1):990. Doi: 10.1186/s12864-017-4385-z.
7.2 Contribution to educational program at external Organization
Dr. Yokokura was reappointed as a member of Executive committee of the Summer School for the Girl’s Junior High School and High School, the summer school program held at the National Women’s Education Center.
7.3 Contribution to the Science project for Ryukyus Girls
OIST and University of the Ryukyus have run the Science project for Ryukyus Girls as a joint program since 2014. This program provides opportunity to K7-K12 girls who live in Okinawa Prefecture (1) to expose scientific activities beyond high school levels, (2) to talk about their interest in science and future with college students who are enrolling STEM program and professionals who are in R&D field at academia or at industry, and (3) to receive some guidance to college education in STEM field and beyond. Dr. Yokokura was asked to give a talk in the Science Camp Vol. 9 (originally scheduled on March 21-22, 2020; postponed due to COVID-19 pandemic). In addition, he has also involved in selection of participants and planning activities of the Science Camp Vol. 9.