FY2022 Annual Report
Computational Neuroethology Unit
Assistant Professor Sam Reiter
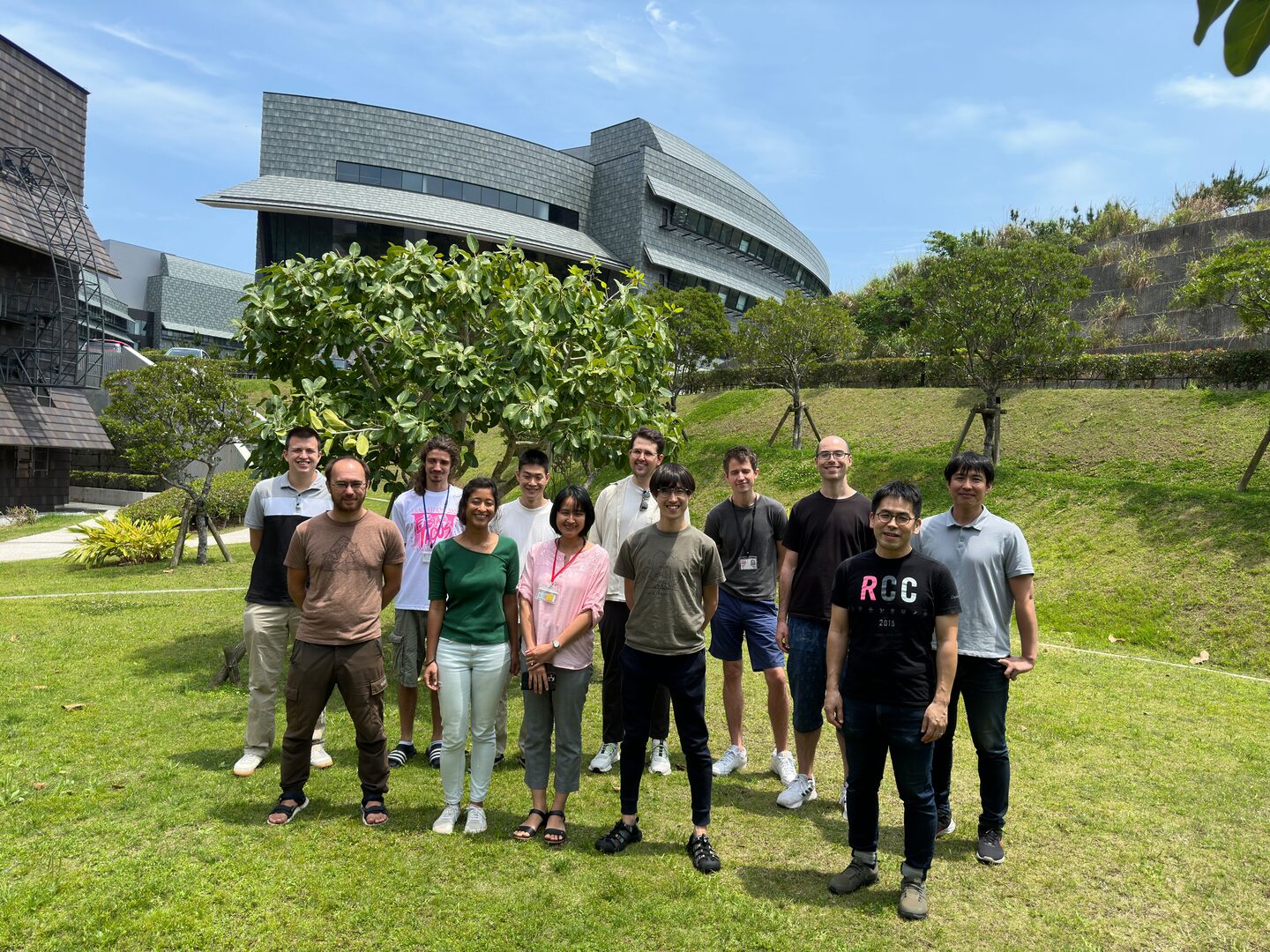
Abstract
The Computational Neuroethology Unit seeks to uncover the principles governing animal behavior and its neural basis. To achieve this goal, the unit combines novel methods for high-resolution behavioral recording with systems neuroscience and computational approaches. The unit currently focuses on coleoid cephalopods (cuttlefish, octopus, and squid), a group of marine invertebrates that evolved uniquely large brains and complex behaviors.
1. Staff
- Dr. Natacha Roux, JSPS Postdoctoral Fellow
- Dr. Tomo Mano, JSPS Postdoctoral Fellow
- Dr. Makoto Hiroi, Staff Scientist (with Stephens Unit)
- Dr. Robert Ross, Interdisciplinary Postdoctoral Fellow (with Pigolotti Unit)
- Dr. Alex Harston, JSPS Postdoctoral Fellow
- Dr. Nobuaki Mizumoto, Interdisciplinary Postdoctoral Fellow (with Bourguignon Unit)
- Ms. Shoko Yamakawa, Research Unit Administrator
- Ms. Van Dinh, Laboratory Technician
- Mr. Keishu Asada, Fieldwork Technician
- Mr. Yutaka Kojima, Laboratory Technician
- Ms. Aditi Pophale, Graduate Student
- Mr. Kostas Tsaridis, Graduate Student
- Mr. Ethan Thomas Rickards, Rotation Student
- Mr. Olivier Fernandez, Intern Student
2. Collaborations
2.1 Squid collective behavior
- Description: Decoding the language of social cephalopods.
- Type of collaboration: Joint research
- Researchers:
- Professor Greg Stephens, Vrije Universiteit Amsterdam/OIST
- Dr. Makoto Hiroi, OIST
2.2 Cephalopod Physiology
- Description: Analysis of neural recordings from cephalopod brains
- Type of collaboration: Joint research
- Researchers:
- Dr. Leenoy Meshulam, University of Washington
2.3 Cephalopod skin as growing active matter
- Description: Analysis of skin pattern growth and development
- Type of collaboration: Joint research
- Researchers:
- Professor Simone Pigolotti, OIST
- Dr. Robert Ross, OIST
2.4 Cuttlefish Camouflage Dynamics
- Description: Describing the space of cuttlefish camouflage, and how they move in this space
- Type of collaboration: Joint research
- Researchers:
- Professor Gilles Laurent, MPI for Brain Research
- Ms. Theodosia Woo, MPI for Brain Research
- Dr. Xitong Liang, MPI for Brain Research
- Dr. Dominic Evans, MPI for Brain Research
2.5 Clownfish behavior
- Description: Tracking clownfish social behavior in 4 dimensions
- Type of collaboration: Joint research
- Researchers:
- Professor Vincent Laudet, OIST
- Dr. Manon Mercader, OIST
2.6 Termite behavior
- Description: Tracking termite tandem running
- Type of collaboration: Joint research
- Researchers:
- Professor Tom Bourguignon, OIST
- Dr. Nobuaki Mizumoto, OIST
2.7 Cephalopod Genetics
- Description: genomics and transcriptomics
- Type of collaboration: Joint research
- Researchers:
- Professor Dan Rokhsar, OIST
- Dr. Gustavo Sanchez, OIST
2.8 Octopus chemosensation
- Description: from molecules to behavior
- Type of collaboration: Joint research
- Researchers:
- Professor Nick Bellono, Harvard University
- Dr. Rebecka Sepela, Harvard University
- Dr. Pablo Villar del Rio, Harvard University
3. Activities and Findings
From OIST press release: https://www.oist.jp/news-center/news/2023/6/29/cuttlefish-camouflage-more-meets-eye
Cuttlefish, along with other cephalopods like octopus and squid, are masters of disguise, changing their skin color and texture to blend in with their underwater surroundings.
Now, in a study published 28 June in Nature, researchers at the Okinawa Institute of Science and Technology (OIST) and the Max Planck Institute for Brain Research have shown that the way cuttlefish generate their camouflage pattern is much more complex than previously believed.
Cuttlefish create their dazzling skin patterns by precisely controlling millions of tiny skin pigment cells, called chromatophores. Each chromatophore is surrounded by a set of muscles, which contract and relax under direct control of neurons in the brain. When the muscles contract, the pigment cell is expanded and when they relax, the pigment cell is hidden. Together, the chromatophores act like cellular pixels to generate the overall skin pattern.
Professor Sam Reiter, who leads the Computational Neuroethology Unit at OIST said: “Prior research suggested that cuttlefish only had a limited selection of pattern components that they would use to achieve the best match against the environment. But our latest research has shown that their camouflaging response is much more complicated and flexible – we just hadn’t been able to detect it as previous approaches were not as detailed or quantitative.”
Data from around 200,000 skin pattern images were then crunched by the supercomputer at OIST and analyzed by a type of artificial intelligence, known as a neural network. The neural network looked holistically at the different elements of the skin pattern images, including roughness, brightness, structure, shape, contrast, and more complex image features. Each pattern was then placed into a specific location in ‘skin pattern space’, a term the scientists coined to describe the full spectrum of skin patterns generated by the cuttlefish.
The researchers also used the same process to analyze images of the background environment, and looked at how well the skin patterns matched the environment.
Overall, the researchers found that the cuttlefish were able to display a rich variety of skin patterns and could sensitively and flexibly change their skin pattern to match both natural and artificial backgrounds. When the same animal was presented with the same background multiple times, the resulting skin patterns subtly differed in ways that were indistinguishable to the human eye.
The path that the cuttlefish took to reach each skin pattern was indirect. The cuttlefish transitioned through a range of different skin patterns, pausing in between, with each pattern change improving the camouflage until the cuttlefish stabilized on a pattern they seemed satisfied with. Such paths, even between the same two backgrounds, were never the same, emphasizing the complexity of the cuttlefish’s behavior.
“The cuttlefish would often overshoot their target skin pattern, pause, and then come back,” said Theodosia Woo, joint first author of the study and graduate student in the Max Planck Institute for Brain Research team. “In other words, cuttlefish don’t simply detect the background and go straight to a set pattern, instead, it is likely that they continuously receive feedback about their skin pattern and use it to adjust their camouflage. Exactly how they receive that feedback – whether they use their eyes, or whether they have a sense of how contracted the muscles around each chromatophore are – we don’t yet know.”
The researchers also examined another skin pattern display, called blanching, which occurs when cuttlefish turn pale in response to a threat. “Unlike camouflaging, blanching was fast and direct, suggesting it uses a different and repeatable control system,” said Dr. Dominic Evans, a postdoctoral fellow in the Max Planck Institute for Brain Research team.
When the researchers took high resolution images of the blanching display, they realized that some elements of the previous camouflage pattern remained, with the blanching pattern superimposed on top. Afterwards, the cuttlefish would slowly but reliably return to displaying its pre-blanching skin pattern.
“This suggests that information about the initial camouflage somehow remains. The blanching is more like a response that temporarily overrides the camouflage signals from the brain and might be controlled by a completely different neural circuit in the brain,” explained Dr. Xitong Liang, joint first author of the study and former postdoctoral researcher in the Max Planck Institute for Brain Research team. “The next step is to capture neural recordings from cuttlefish brains, so we can further understand exactly how they control their unique and fascinating skin patterning abilities.”
4. Publications
4.1 Journals
Nakajima R, Lajbner Z, Kuba MJ, Gutnick T, Iglesias TL, Asada K, Nishibayashi T, Miller J. (2022) Squid adjust their body color according to substrate, Scientific Reports 12(1):5227.
4.2 Books and other one-time publications
Nothing to report
4.3 Oral and Poster Presentations
- Reiter S., Tokyo University. Cephalopod Behavior part 1, Tokyo, Japan.
- Reiter S., Tokyo University. Cephalopod Behavior part 2, Tokyo, Japan.
- Reiter S., Neural Dynamics across Species' symposium, MPI for Brain Research. Exploring 2 stage sleep in octopus, Frankfurt, Germany.
- Reiter S., Cajal Summer Course on Quantiative Animal Behavior 2022. Cephalopod Behavior, Champalimaud, Lisbon, Portugal.
- Meshulam L., Pophale A., Shimizu K., Mano T., Asada K., Reiter S., NEURO2022. Octopus camouflage: a multiscale approach, Okinawa, Japan.
- Mano T., Nambu M. F., Nomura S., Shimizu K., Reiter S., The 45th Annual Meeting of the Japan Neuroscience Society. Whole body/brain imaging of cephalopod nervous system using tissue clearing methods, Okinawa, Japan.
- Shimizu K., Pophale A., Mano T., Meshulam L., Reiter S., International Symposium on Artificial Intelligence and Brain Science. Behavioral and Neural Correlates of Two-stage Sleep in Cephalopods, OIST, Okinawa, Japan.
- Mano T., 10th Plant Imaging Seminar. Whole body/brain imaging of the cephalopod nervous system using tissue clearing methods, OIST, Okinawa, Japan.
- Reiter S., East Asia Joint Symposium on Biomedical Research. Atlas construction in non model brains.
- Reiter S., Riken CBS. Exploring 2-stage sleep in octopus.
- Reiter S., IEEE Humanoids conference. Evolutionary convergence of complex cognition.
- Mano T., 7th ABiS Advanced Light Microscopy Course. Whole body/brain imaging of the cephalopod nervous system, OIST, Okinawa, Japan.
- Meshulam L., Pophale A., Shimizu K., Mano T., Reiter S., American Physicial Society March Meeting. From 100K pigment cells to skillful camouflage: the role of skin color changes in sleeping octopuses, Las Vegas, Nevada, USA.
- Reiter S., The 46th Annual Meeting of the Japan Neuroscience Society. Wake-like skin patterning and neural activity during octopus sleep.
- Reiter S., Institute of Behavioural Neuroscience. Wake-like skin patterning and neural activity during octopus sleep, UCL, London UK.
- Asada K., Chanyoung K., Lajbner Z., Iglesias T. L. , and Reiter S., CIAC 2022 (Cephalopod International Advisory Council. Raising small intertidal octopus Octopus incella Kaneko & Kubodera, 2007 from egg to maturity, Sesimbra, Portugal.
- Mano T., Mambu M. F., Reiter S., NEURO2022. Whole body/brain imaging of cephalopod nervous system using tissue clearing methods, Okinawa, Japan.
- Asada K., Chanyoung K., Lajbner Z., Iglesias T. L., and Reiter S., 7th Okinawa Marine Science Workshop. Raising small intertidal octopus Octopus incella Kaneko & Kubodera, 2007 from egg to maturity, University of the Ryukyus, Okinawa, Japan.
- Mano T., Pophale A., Shimizu K., Iglesias T., Martin K., Hiroi M., Asada K., Andaluz P. G., Dinh T.T.V., Meshulam L., Reiter S., COSYNE 2023. Wake-like Skin Patterning and Neural Activity During Octopus Sleep, Montreal, Canada.
5. Intellectual Property Rights and Other Specific Achievements
Nothing to report
6. Meetings and Events
Nothing to report